Nanoparticules d'or multifonctionnelles pour des applications diagnostiques et thérapeutiques améliorées :une revue
Résumé
Les propriétés médicales des métaux ont été explorées pendant des siècles en médecine traditionnelle pour le traitement des infections et des maladies et sont encore pratiquées à ce jour. Les médicaments à base de platine sont la première classe de médicaments à base de métaux à être cliniquement utilisés comme agents anticancéreux après l'approbation du cisplatine par la Food and Drug Administration (FDA) des États-Unis il y a plus de 40 ans. Depuis lors, davantage de métaux bénéfiques pour la santé ont été approuvés pour des essais cliniques. Fait intéressant, lorsque ces métaux sont réduits en nanoparticules métalliques, ils ont présenté des propriétés uniques et nouvelles qui étaient supérieures à leurs homologues en vrac. Les nanoparticules d'or (AuNP) font partie des nanoparticules métalliques approuvées par la FDA et se sont révélées très prometteuses dans divers rôles en médecine. Ils ont été utilisés comme agents d'administration de médicaments, photothermiques (PT), de contraste, thérapeutiques, radiosensibilisants et de transfection génique. Leurs applications biomédicales sont passées en revue ici, couvrant leur utilisation potentielle dans le diagnostic et le traitement des maladies. Certains des systèmes basés sur AuNP qui sont approuvés pour les essais cliniques sont également discutés, ainsi que les menaces potentielles pour la santé des AuNP et certaines stratégies qui peuvent être utilisées pour améliorer leur biocompatibilité. Les études examinées offrent une preuve de principe selon laquelle les systèmes basés sur AuNP pourraient potentiellement être utilisés seuls ou en combinaison avec les systèmes conventionnels pour améliorer leur efficacité.
Introduction
La médecine fait partie des nombreux domaines qui ont bénéficié des nanotechnologies. La nanotechnologie a émergé avec de nombreuses opportunités pour améliorer et développer de nouveaux agents diagnostiques et thérapeutiques grâce à l'utilisation de nanomatériaux [1, 2]. Les AuNPs, en particulier, présentent des propriétés physico-chimiques uniques et une bonne stabilité chimique. Ils sont faciles à fonctionnaliser avec presque tous les types de molécules électrodonneuses, par diverses chimies ou sur la base de leur forte affinité pour les molécules thiolées [3, 4]. En raison de leur petite taille, les AuNPs ont une plus grande surface et une capacité de charge médicamenteuse élevée. De multiples fractions peuvent être incorporées dans les AuNP pour des applications biomédicales ; ceux-ci incluent des molécules de ciblage pour augmenter la spécificité, des agents de contraste pour la bio-imagerie et pour surveiller la réponse de la maladie aux médicaments en temps réel, et des agents thérapeutiques pour le traitement de la maladie [5, 6]. Fait intéressant, même sans aucune biomolécule ajoutée, les AuNP sont capables de cibler, d'imager et de traiter des maladies. Sur la base de leurs propriétés dépendantes de la taille, de nouveaux systèmes basés sur AuNP peuvent être créés pour une utilisation dans diverses applications biomédicales [7].
Les AuNPs sont fabriqués à partir d'un précurseur métallique qui est thermostable et sont donc très stables et non biodégradables. L'or en vrac est utilisé en médecine et s'est avéré être bio-inerte et non toxique [8, 9]; par conséquent, le noyau d'or dans les AuNPs affichera essentiellement des propriétés similaires [3, 10]. Les AuNP et leurs applications ont été largement étudiées depuis plus de cinq décennies et se sont révélées très prometteuses en tant qu'agents théranostiques dans les études précliniques [5, 11,12,13] et cliniques [14,15,16,17,18]. De nombreuses autres opportunités pour de nouveaux systèmes basés sur AuNP existent, comme discuté dans cette revue. Les AuNP sont déjà explorées dans des essais cliniques en tant que transporteurs de médicaments pour le traitement des cancers à un stade avancé [16, 17] et en tant qu'agents PT dans le traitement du cancer de la prostate [19] et de l'acné [18]. Sans remettre en cause les problèmes de santé et de réglementation qui entourent l'utilisation des AuNPs [20], un avenir pour ces systèmes en biomédecine est à portée de main. Des systèmes multifonctionnels à base d'AuNP capables de lutter contre la résistance aux médicaments avec une efficacité localisée et améliorée sont possibles [11, 21, 22]. La revue met en évidence les propriétés biologiques des AuNP dans les études précliniques et cliniques, en réfléchissant à leurs bio-applications en tant qu'agents diagnostiques et thérapeutiques. Leurs menaces potentielles pour la santé et les stratégies utilisées pour surmonter leurs limites sont également décrites. Enfin, les perspectives d'avenir des AuNP en médecine sont mises en évidence.
Nanoparticules d'or
La popularité des AuNP dans les applications médicales a pris beaucoup d'ampleur en raison de leurs propriétés chimiques et physiques uniques. Les AuNP sont des particules colloïdales solides dont la taille varie de 1 à 100 nm [23]. Les applications des AuNPs en biologie sont enracinées dans leurs propriétés physico-chimiques, non limitées à leur taille, leur résonance plasmonique de surface (SPR), leur forme et leur chimie de surface [3, 10]. Ces paramètres influencent leur activité et en font des candidats parfaits pour une utilisation dans le diagnostic et le traitement des maladies, que ce soit en tant qu'agents d'administration, de sensibilisation, de contraste ou thérapeutiques. Leur petite taille est associée à une plus grande surface, ce qui permet la modification de surface et la fixation de plusieurs charges utiles, telles que le ciblage, l'imagerie et les agents thérapeutiques [4, 24, 25, 26]. Leur petite taille permet également aux NP et à leur cargaison de traverser des barrières biologiques qui seraient autrement difficiles à atteindre et à pénétrer [11].
Les AuNP sont de plus en plus reconnues comme des agents diagnostiques, thérapeutiques et théranostiques (un agent qui peuvent être utilisés simultanément pour diagnostiquer et traiter une maladie), qui ont le potentiel de traiter les effets hors cible associés aux thérapies conventionnelles. Cependant, les AuNPs possèdent des propriétés et des fonctions différentes par rapport à leurs homologues en vrac biocompatibles, ce qui pourrait être dangereux pour la santé humaine [27,28,29]. L'utilisation clinique de composés d'or en vrac pour le traitement de maladies est une pratique ancienne et certifiée sûre [8]. Ces dernières années, des recherches ont montré que les AuNP ont des propriétés médicales similaires ou améliorées [29]. En raison de leurs propriétés optiques, chimiques et physiques uniques, les AuNP présentent souvent de nouvelles propriétés par rapport à l'or en vrac [30, 31] et peuvent servir d'agents diagnostiques et thérapeutiques [5].
Synthèse des AuNP
Les AuNP peuvent être produites de plusieurs manières suivant l'approche descendante ou ascendante. L'approche descendante utilise des méthodes physiques et chimiques pour produire les tailles souhaitées à partir du matériau en vrac, tandis que l'approche ascendante implique des méthodes chimiques pour assembler les éléments constitutifs de la formation de systèmes nanométriques [32, 33]. Les méthodes physiques (telles que le broyage, la photochimie, le rayonnement et la lithographie) utilisent une énergie et une pression considérables pour réduire les matériaux en vrac en 10 –9 milliardième de mètre [10, 32, 34]. Les processus de nucléation sont facilement contrôlés lors de l'utilisation des méthodes physiques, les agents réducteurs ne sont pas nécessaires, et avec certaines de ces méthodes, la synthèse se produit simultanément avec la stérilisation des NP. Cependant, les technologies physiques sont souvent coûteuses, difficilement disponibles et nécessitent un équipement spécialisé. De plus, les agents de coiffage et de stabilisation peuvent ne pas survivre aux processus à haute énergie impliqués dans ces processus [34].
L'approche ascendante est principalement préférée dans la synthèse des AuNPs car elle est rapide, facile et ne nécessite pas l'utilisation d'équipements sophistiqués [33,34,35]. Il est basé sur la méthode chimique développée par Turkevich en 1951 (Fig. 1A), qui utilise du citrate pour la réduction et la stabilisation d'un précurseur d'or, résultant en la production d'AuNPs sphériques de 15 nm [3, 10, 23, 33, 36 , 37]. La méthode a été encore modifiée en faisant varier le rapport entre la teneur en citrate et en précurseur d'or et a abouti à une plage de diamètres de taille de 15 à 150 nm AuNPs (Fig. 1B) [10, 24]. Un certain nombre d'agents réducteurs tels que le borohydrure de sodium, le bromure de cétyltriméthylammonium (CTAB) et l'acide ascorbique ont également été introduits. Certains des agents réducteurs chimiques sont malheureusement toxiques [33, 34, 36] et généralement passivés en ajoutant à leur surface des agents stabilisants tels que le polyéthylène glycol (PEG), la gomme arabique, les polysaccharides et les peptides bioactifs [37, 38].
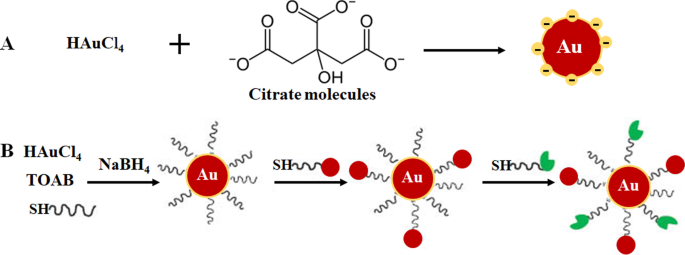
Formulation d'AuNP via un système monophasique par réduction de citrate (A ) et réduction du système en deux phases suivie d'une stabilisation et d'une fonctionnalisation via une réaction d'échange de ligand, méthode Brust-Schiffrin (B ). Reproduit avec permission [36]. Copyright 2013, De Gruyter. TOAB bromure de tétrabutylammonium, SH molécules thiolées
Des approches plus écologiques telles que le procédé plasma-in-liquide induit par micro-ondes (MWPLP) et la nanotechnologie verte ont été explorées dans les AuNPs de synthèse pour éviter l'utilisation d'agents réducteurs chimiques toxiques. Le MWPLP utilise des micro-ondes pour générer la nucléation des NP métalliques et ne nécessite aucun agent réducteur, et l'énergie requise pour la synthèse est très faible [34]. La nanotechnologie verte, quant à elle, utilise des composés naturels provenant de plantes et de micro-organismes comme source d'agents réducteurs dans la synthèse d'AuNPs biogènes [12, 33, 39, 40, 41]. La nanotechnologie verte est considérée comme écologique et respectueuse de l'environnement et donc plus adaptée aux applications biomédicales. La synthèse à médiation végétale est plus économique que l'utilisation de micro-organismes. De plus, la synthèse peut être effectuée en une seule étape et les NP sont plus faciles à purifier. De plus, les plantes sont renouvelables; diverses parties des plantes telles que les feuilles, les tiges, les écorces, les racines, les fleurs et les fruits peuvent être récoltées sans tuer la plante et utilisées pour la synthèse. Les extraits préparés à partir de la matière végétale contiennent des composés phytochimiques, des protéines et des enzymes qui peuvent fonctionner comme agents réducteurs, stabilisants et coiffants [10, 12, 24, 34, 35, 40, 42]. L'épigallocatéchine des thés verts [42] et la mangiférine (MGF) des mangues [12, 43] font partie des composés dérivés de plantes qui ont été largement utilisés pour synthétiser les AuNP [34]. Plus d'informations sur ces méthodes sont examinées en détail dans les références suivantes [10, 24, 34, 35].
Application biologique des AuNP
Le rôle et l'importance des AuNP dans la science médicale deviennent sans aucun doute de plus en plus visibles, ce qui est soutenu par le nombre croissant d'études démontrant leur application multiforme dans un large éventail de domaines biomédicaux. La biocompatibilité des AuNPs est attribuée à la longue histoire de l'or dans le traitement des maladies humaines, qui remonte à 2500-2600 av. Les Chinois et les Indiens utilisaient l'or pour le traitement de l'impuissance masculine, de l'épilepsie, de la syphilis, des maladies rhumatismales et de la tuberculose. La Chine a découvert l'effet de longévité de l'or colloïdal rouge, qui est encore pratiqué en Inde dans le cadre de la médecine ayurvédique pour le rajeunissement et la revitalisation. Le cinabre-or (également connu sous le nom de Makaradhwaja) est utilisé pour améliorer la fertilité en Inde. Dans les pays occidentaux, l'or a été utilisé pour traiter les troubles nerveux et l'épilepsie. Aucune toxicité n'a été signalée pour son utilisation dans les études in vitro et in vivo [8, 44, 45]. Depuis lors, les composés oraux oraux et injectables ont continué à être utilisés comme traitements de l'arthrite [9, 46] et il a également été démontré qu'ils avaient des effets anticancéreux [8]. Des effets similaires et dans certains cas améliorés ont également été signalés pour les AuNP, qui apparaissent comme des agents prometteurs pour le diagnostic de la maladie [47,48,49] et la thérapie [3, 29, 50, 51].
Les AuNPs ont une plus grande surface qui peut être exploitée pour des applications biomédicales, en attachant diverses biomolécules pour répondre à une fonction souhaitée. Ceux-ci peuvent inclure des fragments de ciblage pour aider à reconnaître des biomarqueurs spécifiques à la maladie, des agents de contraste pour la bio-imagerie et des agents thérapeutiques pour le traitement des maladies [24, 25]. L'avantage de l'utilisation des AuNP par rapport à d'autres nanomatériaux est qu'ils peuvent être facilement fonctionnalisés à l'aide de diverses chimies, comme le montre la figure 2 [4, 26]. Les AuNPs ont une grande affinité pour les molécules thiolées, et la liaison thiol-or est la méthode la plus couramment utilisée pour adsorber les molécules sur la surface des NP [4]. Des chimies basées sur l'affinité telles que la liaison biotine-streptavidine et le couplage carbodiimide sont également utilisées. Les AuNP sont utilisées dans trois domaines principaux de la biomédecine :la délivrance de produits pharmaceutiques, à des fins diagnostiques et thérapeutiques [24, 35], et ont démontré un énorme potentiel dans ces domaines, comme indiqué ci-dessous.
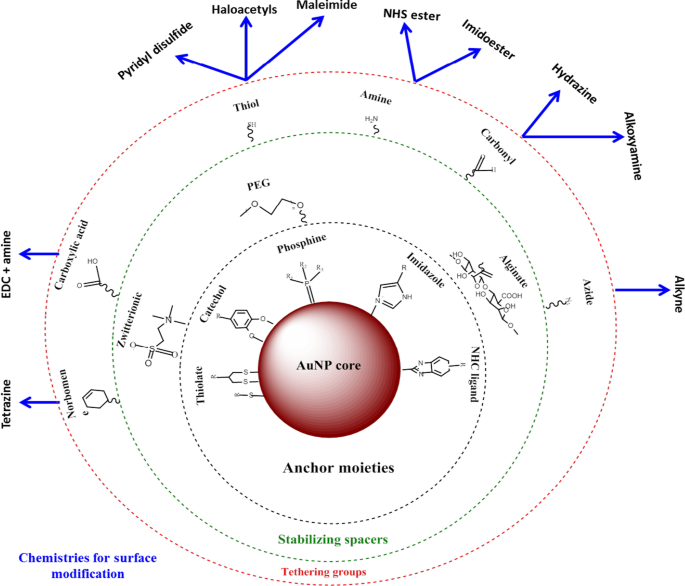
Synthèse et fonctionnalisation des AuNPs. Les biomolécules avec des groupes fonctionnels sont d'abord adsorbées sur la surface de la NP par affinité or-thiol. Ensuite, d'autres groupes fonctionnels tels qu'un groupe amine peuvent être utilisés pour lier des molécules avec des groupes carboxyle pour attacher des fragments de ciblage ou de médicament. Adapté de [32]
AuNP en tant qu'agents d'administration de médicaments
L'application la plus courante des AuNP est en tant que véhicules d'administration de médicaments [11, 18, 52], de vaccins [53] et de thérapie génique [24, 32]. Les AuNP possèdent des propriétés qui peuvent résoudre la plupart des problèmes associés aux thérapies conventionnelles telles que la résistance aux médicaments, la faible distribution des médicaments, la biodégradation et la clairance précoce des médicaments [11]. Les AuNP peuvent réduire considérablement le dosage des médicaments, la fréquence des traitements et sont capables de transporter des médicaments hydrophobes et insolubles. Ils sont considérés comme bio-inertes et peuvent masquer leur cargaison des attaques des cellules immunitaires, protéger les médicaments de la dégradation protéolytique lors de leur passage dans le système circulatoire et ainsi augmenter le temps de circulation des médicaments. Ces facteurs peuvent facilement augmenter l'efficacité des médicaments en les concentrant et en les retenant dans les tissus malades avec peu ou pas d'effet sur les tissus normaux [25].
L'utilisation des AuNPs dans le traitement du cancer a été largement étudiée [17, 37, 54], et au fil des ans, elle a été étendue à d'autres maladies telles que l'obésité [50, 55, 56] et l'acné [18]. Les systèmes à base de nano sont plus petits que la plupart des composants cellulaires et peuvent traverser passivement les barrières cellulaires en tirant parti de l'effet amélioré de perméabilité et de rétention (EPR) sur le système vasculaire des tissus malades [25]. L'EPR dans un état pathologique est caractérisé par une angiogenèse excessive et une sécrétion accrue de médiateurs de perméabilité, qui peuvent augmenter l'absorption d'AuNP par les tissus malades. Ces caractéristiques ne sont associées qu'à des états pathologiques et non à des tissus normaux, ce qui permet un ciblage sélectif des conjugués AuNP [25]. Les AuNP sont attrayantes en tant que vecteurs de médicaments car elles peuvent transporter plusieurs molécules simultanément, diversifiant davantage leurs propriétés. Il s'agit d'un trait souhaitable en médecine dans lequel la plupart des bio-applications AuNP sont enracinées, car les AuNP peuvent être adaptés pour une fonction biomédicale spécifique. Cela peut aider à contrôler la façon dont ils interagissent avec les organites cellulaires et donc prometteur pour le développement futur de modalités de diagnostic et de traitement efficaces pour diverses maladies [4].
Systèmes de diagnostic basés sur AuNP
L'émergence des nanotechnologies a permis de développer des systèmes de détection rapides, robustes, sensibles et hautement compétitifs par rapport aux tests de diagnostic conventionnels [48]. Les nanomatériaux sont généralement intégrés dans les plateformes de biodétection existantes pour la détection de marqueurs gazeux, ADN et protéiques impliqués dans le développement de maladies [47]. Parmi les divers nanomatériaux (qui comprennent les NP métalliques, polymères, magnétiques et semi-conducteurs) utilisés dans le diagnostic, les AuNP ont été largement utilisés dans les biocapteurs, les capteurs électrochimiques et les tests chromogènes pour détecter ou détecter la présence de biomarqueurs de maladies [49]. Leur SPR localisé (LSPR), leur transfert d'énergie par résonance de fluorescence (FRET), leur diffusion Raman améliorée en surface, leur conductivité, leur activité redox et leur effet de charge quantifié en font un outil idéal pour l'imagerie et la détection de molécules cibles [10, 24]. Leurs propriétés électroniques et optiques, et leur capacité à diffuser la lumière visible et proche infrarouge (NIR) sont compatibles et mesurables avec diverses technologies telles que les techniques microscopiques (diffusion de la lumière électronique, confocale et en champ sombre) [57], tomodensitométrie (CT) , technique d'imagerie PT hétérodyne, spectroscopie UV-Vis et Raman [24, 35].
Le développement de systèmes de diagnostic basés sur AuNP implique la modification de la surface AuNP, par exemple, par la fixation de biomolécules qui reconnaissent les biomarqueurs de la maladie [3, 24, 58]. Les analyses de flux latéral (LFA) sont probablement l'exemple le plus connu d'outils de diagnostic basés sur la nanotechnologie. Les LFA utilisent généralement des AuNP d'environ 30 à 40 nm, car les particules plus petites ont une très petite section efficace d'extinction, tandis que les particules plus grosses sont généralement instables pour une utilisation dans ces tests [59]. De plus, d'autres molécules/enzymes pouvant déclencher des changements dans la SPR, la conductivité et l'oxydoréduction des AuNP sont incluses. Ces indicateurs donnent un signal détectable après liaison des analytes aux conjugués AuNP [24], l'absence ou la présence de signal reflétera alors l'absence ou la présence de la molécule cible ou de la maladie. Le signal généré par les AuNPs est chimiquement stable, durable et cohérent lorsqu'il est utilisé dans différents formats de test :tube à essai, bandelette, in vitro et in vivo [24]. Par conséquent, leur application a remarquablement augmenté la vitesse et le succès des tests de diagnostic.
Dosages colorimétriques basés sur AuNP
Dans les dosages colorimétriques, les AuNP produisent un signal visuel (généralement un changement de couleur) qui peut être détecté à l'œil nu sans l'utilisation d'instruments avancés. Généralement, une solution colloïdale d'AuNPs a une couleur rouge rubis à raisin qui dépend fortement de la distance interparticulaire [60, 61]. La liaison d'un analyte aux AuNPs modifiés avec des éléments de bio-reconnaissance moléculaire (par exemple, des anticorps, des peptides, des aptamères, des enzymes, etc.) induit un changement distinct dans le LSPR, entraînant par conséquent le changement de couleur du rouge rubis au bleu [60 , 62, 63]. L'intensité de la couleur est directement proportionnelle à la concentration d'un analyte et sert à confirmer la présence et l'état de la maladie. Le diagnostic colorimétrique basé sur AuNP a été utilisé avec succès dans la détection du virus de la grippe A [64], du virus Zika [65], du bactériophage T7 [66], de Mycobacterium tuberculosis [67], et récemment, pour la détection du syndrome respiratoire aigu sévère-coronavirus-2 (SARS-CoV-2) [60, 68].
Un exemple d'un test colorimétrique basé sur AuNP a été démontré pour la détection du SRAS-CoV-2 [60], un virus qui provoque une maladie à virus Corona 2019 (COVID-19) hautement infectieuse [60, 68]. Avec ce dosage, la présence du virus a été signalée par un simple changement de couleur; aucune instrumentation n'a été nécessaire pour faire le diagnostic. Les tests de diagnostic clinique actuels de ce virus utilisent soit le test d'amplification en chaîne par polymérase en temps réel (RT-PCR), qui prend de 4 à 6 heures, tandis que les systèmes de point de service (PoC) rapides détectent les anticorps qui pourraient prendre plusieurs jours pour apparaître dans le sang. En comparaison, le dosage colorimétrique basé sur AuNP était plus robuste et plus rapide, comme le montre la figure 3. L'incubation des AuNPs marqués avec des oligonucléotides antisens (ASO) en présence d'échantillons d'ARN du SRAS-CoV-2 a entraîné la formation d'un précipité bleu dans ~ 10 min. Dans un test positif pour le SRAS-CoV-2, la liaison des ASO au gène N dans la phosphoprotéine de la nucléocapside du virus a induit une couleur bleue qui a été détectée visuellement. Le test était très sensible et avait une limite de détection de 0,18 ng/μL pour l'ARN du SRAS-CoV-2 [60].
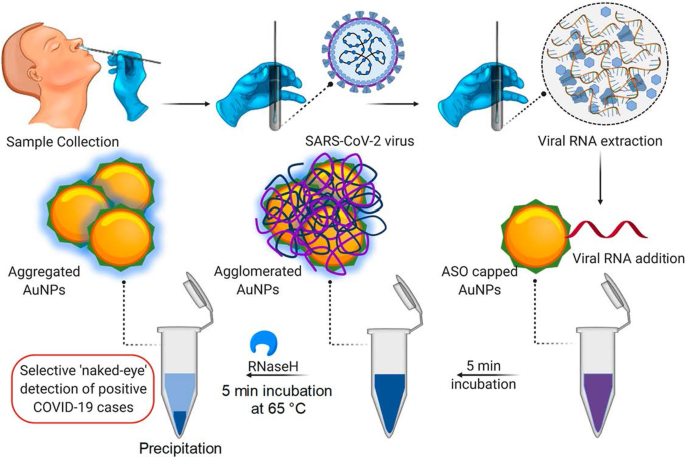
Système de diagnostic colorimétrique basé sur AuNP. Détection sélective à l'œil nu de l'ARN du SRAS-CoV-2 par les AuNPs plafonnés ASO. Reproduit avec permission [60]. Copyright 2020, ACS Nano
Les LFA basés sur AuNP suivent le même principe que celui illustré à la figure 3 ; cependant, au lieu d'un changement de couleur dans une solution, une ligne visible se forme sur une bandelette réactive lorsqu'un analyte est présent. En présence d'un analyte, les AuNP ont été capturés sur la ligne de test et ont formé une ligne rouge distincte, qui a été visualisée à l'œil nu. L'intensité de la raie est déterminée par le nombre d'AuNPs adsorbées [69]. Un exemple d'un LFA simple et rapide basé sur AuNP est montré dans la Fig. 4, pour la détection de Pneumocystis jirovecii (P. jirovecii ) Anticorps IgM dans les sérums humains. Les AuNPs de 40 nm ont été conjugués avec les antigènes synthétiques recombinants (RSA) de P. jirovecii , soit la principale glycoprotéine de surface, soit la sérine protéase de type kexine, qui ont été utilisées comme indicateur de la présence ou de l'absence de P. jirovecii . Dans un test positif, le P. jirovecii L'IgM a été capturée par le conjugué AuNP-RSA au niveau du tampon de conjugué. Le complexe AuNP-RSA/IgM s'écoule ensuite vers la membrane analytique où il se lie aux IgM anti-humaines (ligne de test) et l'excès se déplace vers les anticorps anti-RSA (ligne de contrôle), ce qui entraîne deux lignes rouges. Le test négatif n'aura qu'une couleur rouge sur la ligne de contrôle [70]. Une étude indépendante a utilisé le LFA basé sur AuNP pour détecter sélectivement l'IgM du SRAS-CoV-2, comme le confirme l'apparition des lignes rouges dans les lignes de test et de contrôle [68]. La couleur a été détectée visuellement à l'œil nu dans les 15 minutes dans les deux systèmes, et seuls 10 à 20 μL d'échantillons de sérum ont été nécessaires par test [68, 70].
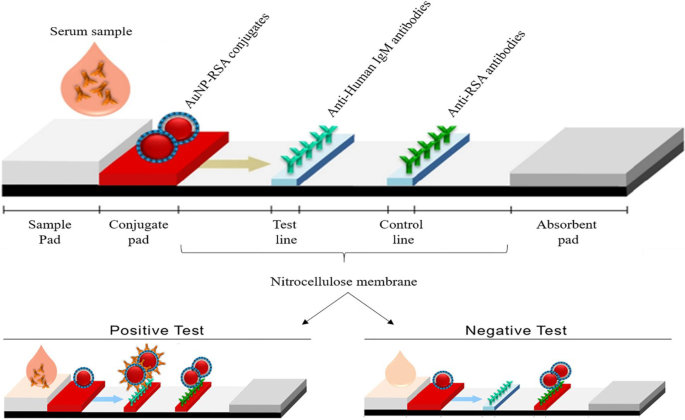
Les LFA basés sur AuNP pour la détection des IgM P. jirovecii anticorps. La présence (test positif) ou l'absence (contrôle négatif) du P. jirovecii les anticorps pouvaient être différenciés par la couleur rougeâtre AuNP dans les deux lignes de test et de contrôle, ou uniquement dans la ligne de contrôle, respectivement. Reproduit avec permission [70]. Copyright 2019, Frontières en microbiologie
L'un des premiers exemples d'utilisation d'AuNPs comme sonde de signalisation sur un LFA était pour la détection de cellules Ramos; l'aptamère TE02 a été utilisé comme sonde de capture et l'aptamère TD05 comme sonde de détection. Le biocapteur aptamère-AuNP peut détecter visuellement un minimum de 4 000 cellules Ramos sans aucune instrumentation et 800 cellules Ramos avec un lecteur de bandelettes portable en 15 min. À l'aide de ce biocapteur de détection en sandwich, le test a détecté avec succès des cellules Ramos dopées dans le sang humain [71] et a été utilisé comme preuve de concept pour développer des systèmes rapides, sensibles et peu coûteux pour la détection qualitative et quantitative des cellules cancéreuses circulantes. Depuis lors, divers LFA basés sur AuNP ont été conçus pour le diagnostic de nombreuses maladies infectieuses, y compris les maladies causées par Pneumocystis pneumonia [70], virus Ebola [72], VIH, virus de l'hépatite C et Mycobacterium tuberculosis [73] et plus récemment le virus SARS-CoV-2 [68].
Systèmes d'imagerie basés sur AuNP
Les AuNP ont été intensivement étudiées pour des applications en bio-imagerie en raison de leur capacité à absorber et à diffuser la lumière correspondant à leurs longueurs d'onde de résonance, jusqu'à 10 5 fois plus que les fluorophores conventionnels [74]. Les AuNP ont un numéro atomique et une densité électronique plus élevés (79 et 19,32 g/cm 3 ) par rapport aux agents conventionnels à base d'iode (53 et 4,9 g/cm 3 ), s'avérant ainsi de meilleurs agents de contraste [24]. Les AuNP s'accumulent sur les cellules ou les tissus malades et induisent une forte atténuation des rayons X, rendant le site ciblé très distinct et facilement détectable. Les AuNP sont attachées à des fragments chimiques et à des agents de bio-reconnaissance moléculaire qui peuvent cibler sélectivement des antigènes spécifiques pour induire un contraste distinct et spécifique à la cible pour l'imagerie CT [75].
Un système d'imagerie CT moléculaire ciblé in vitro a été réalisé en utilisant des AuNPs fonctionnalisés avec un aptamère d'ARN qui se lie à l'antigène membranaire spécifique de la prostate (PSMA). Le conjugué d'aptamère AuNP-PSMA a montré une intensité CT plus de quatre fois supérieure pour les cellules prostatiques exprimant PSMA (LNCaP) par rapport aux cellules prostatiques PC-3, qui manquent du récepteur cible [76]. De même, le conjugué AuNP-acide diatrizoïque-aptamère AS1411 a été localisé dans des cellules CL1-5 (adénocarcinome pulmonaire humain) et des souris porteuses de tumeurs CL1-5. L'aptamère AS1411 cible le récepteur de la nucléoline (NCL) qui est exprimé par les cellules CL1-5 à la surface cellulaire, tandis que l'acide diatrizoïque est un agent de contraste à base d'iode. Le conjugué AuNP-acide diatrizoïque-aptamère AS1411 avait une courbe d'atténuation linéaire avec une pente de 0,027 mM Au unité Hounsfield (HU −1 ) indiquant une accumulation des AuNPs au niveau du site tumoral [77]. Les AuNPs présentaient un temps de rétention vasculaire plus long, ce qui prolongeait leur temps de circulation dans le sang [77,78,79] et améliorait le signal CT de l'acide diatrizoïque [77].
La figure 5 montre une imagerie vasculaire CT in vivo des artères coronaires à l'aide d'AuNP conjugués à la protéine d'adhésion liant le collagène 35 (CNA35) pour cibler le collagène I dans l'infarctus du myocarde chez les rongeurs. Le signal AuNP était toujours détecté dans le sang 6 h après l'administration intraveineuse (i.v), ce qui était significativement plus élevé que la demi-vie (5 à 10 min) des agents à base d'iode [79]. Ces effets ont été reproduits en utilisant des AuNPs coiffées de mannane synthétisées en vert, qui ont montré une absorption médiée par les récepteurs et une non-toxicité dans les cellules exprimant le mannose (DC 2.4 et RAW 264.7). Les AuNPs coiffées de mannane ciblaient sélectivement les ganglions lymphatiques poplités in vivo après injection dans la patte arrière des souris [38]. L'imagerie CT basée sur AuNP peut fournir des informations importantes pour le diagnostic de diverses maladies non limitées à l'artère coronaire et aux cancers [76, 77, 79, 80, 81]. L'utilisation d'AuNPs comme agents de contraste a montré un potentiel dans d'autres systèmes d'imagerie tels que l'imagerie photoacoustique, nucléaire, l'échographie et l'imagerie par résonance magnétique. Ces systèmes sont largement examinés ailleurs [82, 83].
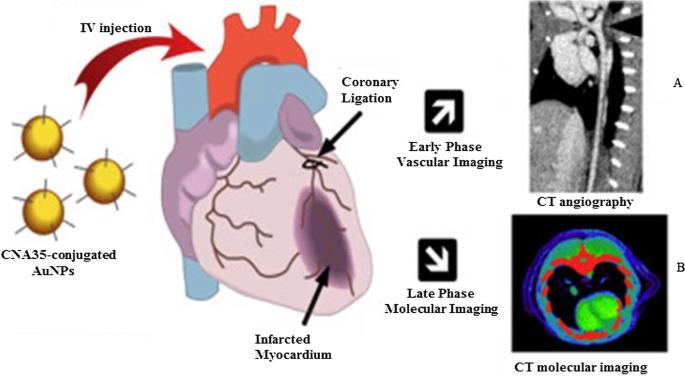
Imagerie CT in vivo utilisant des AuNPs comme agents de contraste CT. Les AuNPs coiffées de Mannan et leur imagerie CT du ganglion lymphatique (A ) et imagerie CT AuNPs conjuguée à CNA35 de la charge de cicatrice myocardique (B ). Reproduit avec permission [79]. Copyright 2018, Elsevier
AuNPs dans les systèmes de détection fluorescents
Les AuNP sont utilisées dans les systèmes de détection à base de fluorescence en tant qu'agents fluorescents ou extincteurs fluorescents. À des tailles 5 nm, les AuNP affichent les propriétés des points quantiques (QD) et peuvent être utilisés à leur place. L'Au55 (PPh3 )12 Cl6 Les nanoclusters introduits en 1981 sont probablement les plus étudiés en raison de leur comportement quantique [7]. Depuis lors, divers AuNPs de taille quantique (AuNPsQ) tels que Au25 (SR)18 , Au38 (SR)24 et Au144 (SR)60 [84] ont été principalement étudiés en détection électrochimique car ce sont d'excellents conducteurs électroniques et médiateurs redox [85].
Des électrodes à film AuNPsQ ont été utilisées dans la fabrication d'un immunocapteur électrochimique ultrasensible pour la détection de l'antigène spécifique de la prostate (PSA). L'immunocapteur avait une sensibilité de 31,5 A mL/ng et une limite de détection de 0,5 pg/mL pour le PSA dans 10 L de sérum humain non dilué. Le dosage immunologique a donné des résultats huit fois supérieurs à ceux d'un immunocapteur forestier à nanotubes de carbone précédemment rapporté contenant plusieurs fragments, à une concentration de biomarqueur inférieure aux niveaux associés à la présence d'un cancer. En tant que tel, il peut être utilisé pour mesurer le biomarqueur de test dans les états normaux et pathologiques. Les performances de l'immunocapteur étaient comparables à la méthode ELISA de référence [86]. AuNPsQ a également été incorporé dans CaCO3 poreux structuré sphères pour former un CaCO3 fluorescent Hybride /AuNPsQ pour la détection de l'énolase spécifique des neurones, un biomarqueur diagnostique et pronostique des lésions cérébrales traumatiques et du cancer du poumon. Le capteur avait une limite de détection de 2,0 pg mL −1 [87]. Jusqu'à présent, plusieurs systèmes de détection fluorescente basés sur AuNP ont été rapportés pour la détection d'analytes associés à l'hépatite B [73, 88], la grippe A [89], le cancer [90] et les lésions cardiaques [91].
Les AuNP sont également d'excellents quenchers basés sur le FRET [92]. Leurs propriétés optiques uniques (intensité de signal stable et résistance au photoblanchiment), leur taille et leur capacité à être modifiées en ont fait des sondes attrayantes dans les plates-formes de détection de fluorescence [93, 94]. Les AuNPs plus grandes (≥ 10-100 nm) ont de faibles rendements quantiques qui ne conviennent pas à la détection directe par fluorescence ; cependant, leur capacité à éteindre les colorants fluorescents dans un état d'énergie d'excitation relativement élevé en a fait des inhibiteurs de photoluminescence efficaces [94]. En principe, les nanosondes de fluorescence sont composées d'un fluorophore donneur (colorant ou QDs) et d'un AuNPs accepteur, et lorsqu'elles sont rapprochées, la fluorescence du fluorophore sélectionné est désactivée par les AuNPs [94, 95]. En l'absence de cible indiquée par l'absence de signal fluorescent, la sonde d'acide nucléique s'hybride et forme une structure en boucle qui rapproche étroitement le fluorophore et un extincteur à ses extrémités opposées ; tandis que la liaison de l'analyte à la sonde d'acide nucléique déplace le fluorophore des AuNPs entraînant un signal fluorescent [24, 94, 96]. Profitant des propriétés mentionnées ci-dessus, les AuNPs ont été incorporées dans des balises moléculaires pour la détection in vitro (nanosphères d'or, AuNSs) et in vivo (nanotiges d'or, AuNRs) de l'expression de la matriptase sur les cellules tumorales. The two molecular beacons were composed of a matriptase cleavage site as a linker between the AuNPs and the fluorophores. The AuNS–molecular beacon was constructed with the fluorescein isothiocyanate (FITC), and the AuNR–molecular beacon had a NIR fluorescent dye (mercaptopropionic acid, MPA). In the absence of the target, the AuNSs and AuNRs, respectively, blocked the FITC and MPA fluorescence. Cleavage of either FITC or MPA from the AuNP–molecular beacons in the presence of matriptase exhibited a quantifiable fluorescence signal. The fluorescent signal of the MPA–AuNR–beacon in the nude mice bearing HT-29 tumors lasted for 14 h in the tumor site, while the signal gradually disappeared from the non-tumor site over time [97].
The AuNPs were reported to have comparable or higher fluorescence quenching efficiency than organic quenchers such as 4-((4′-(dimethyl-amino)phenyl)azo)benzoic acid (DABCYL) [94, 98] and Black Hole Quencher-2 [99]. The fluorescence quenching efficiency of 1.4 nm AuNPs was compatible with the four commonly used organic fluorophores (FITC, rhodamine, texas red and Cy5). The fluorescence quenching efficiency of the AuNPs was similar to that of DABCYL, and unlike DABCYL, the AuNPs showed consistency in both low and high salt buffers [98]. In a competitive hybridization assay, 10 nm AuNPs showed superior (> 80%) fluorescence quenching efficiency for Cy3 dye than the commercial Black Hole Quencher-2 (~ 50%). The assay had a limit of detection of 3.8 pM and a detection range coverage from 3.8 pM to 10 nM for miRNA-205 in human serum, and it was able to discriminate between miRNAs with variations in their nucleotide sequence [99]. The competitive sensor arrays were not only sensitive [96, 99] but were able to differentiate between normal and diseased cells, as well as benign and metastatic cancers [96].
AuNP-Based Bio-barcoding Assay
AuNP-based bio-barcoding assay (BCA) technology has become one of the highly specific and ultrasensitive methods for detection of target proteins and nucleic acids up to 5 orders of magnitude than the conventional assays [100]. The assay relies on magnetic microparticle probes, which are functionalized with antibodies that bind to a specific target, and AuNP probes encoded with DNA that recognizes the specific protein target and antibodies. Upon interaction with the target DNA, a sandwich complex between the magnetic microparticle and AuNPs probes is formed. The sandwich is then separated by the magnet followed by thermal dehybridization to release the free bar-code DNA, enabling detection and quantification of the target [101, 102].
The AuNP-based BCA assay was able to detect HIV-1 p24 antigen at levels that was 100–150-fold higher than the conventional ELISA [103]. The detection limit of PSA using these systems was 330 fg/mL [104]. The versatility of AuNPs for the development of a BCA-based platform was further demonstrated by measuring the concentration of amyloid-beta-derived diffusible ligands (ADDLs), a potential Alzheimer's disease (AD) marker found in the cerebrospinal fluid (CSF). ADDL concentrations were consistently higher in the CSF taken from the subjects diagnosed with AD than in non-demented age-matched controls [105]. These results indicate that the universal labeling technology can be improved through the use of AuNPs to provide a rapid and sensitive testing platform for laboratory research and clinical diagnosis.
AuNP-Based Therapies
Metal-based drugs are not new to medicine; in fact, they are inspired by the existing metallic drugs used in clinical treatment of various diseases [9, 106,107,108,109]. The widely studied and clinically used metal-based drugs were derived from platinum (e.g., cisplatin, carboplatin, tetraplatin for treatment of advanced cancers), bismuth (for the treatment of infectious and gastrointestinal diseases), gold (for the treatment of arthritis) and gallium (for the treatment of cancer-related hypercalcemia) [108, 109]. The approval of cisplatin in 1978 by the FDA for the clinical treatment of cancer [107] further inspired research on other metals (such as palladium, ruthenium, rhodium) [32, 106, 110].
Owing to the bioactivities, which included anti-rheumatic, antibacterial and anticancer effects, and the biocompatibility of bulk gold [8, 9, 46, 111], AuNPs are extensively investigated for the treatment of several diseases. AuNPs displayed unique and novel properties that are superior to its bulk counterpart. AuNPs are highly stable and have a distinct SPR, which guides their application in medicine [112], as drug delivery and therapeutic agents. AuNPs have a lot of advantages over the conventional therapy; they have a longer shelf-life and can circulate long enough in the system to reach their targets [25] with [11, 49, 113] or without targeting molecules [14, 15, 24, 25, 114]. AuNPs can provide localized and selective therapeutic effects; some of the areas in which AuNPs were used in therapy are described below.
Therapeutic Effects of Untargeted AuNPs
The as-synthesized (i.e., unmodified or uncapped) AuNPs have been shown to have diverse therapeutic effects against a number of infectious [115, 116], metabolic and chronic diseases [3, 29, 50, 51]. Their antioxidant, anticancer, anti-angiogenic [3, 32], anti-inflammatory [3, 51] and weight loss [29, 50, 112] effects are beneficial for diseases such as cancer, rheumatoid arthritis, macular degeneration and obesity [5, 25, 113, 117]. The above-mentioned diseases are characterized by a leaky vasculature and highly vascularized blood vessels [5, 113], which provides the NPs an easy passage into the diseased tissues and increase the susceptibility of cells to their effects. Through the EPR effect, uncapped AuNPs can passively accumulate in the vasculature of diseased cells or tissues. Hence, AuNPs have been specifically designed to have anti-angiogenic effects in diseases where angiogenesis (the growth and extension of blood vessels from pre-existing blood vessels) spins out of control like cancer, rheumatoid arthritis, macular degeneration and obesity [5, 25, 113, 117]. Targeting and destroying the defective blood vessels prevent oxygen and nutrients from reaching the diseased cells, which results in their death. The pores in the blood vessels at the diseased site (especially in cancer and obesity) are 200–400 nm and can allow materials in this size range to pass from the vasculature into the diseased tissues and cells [14, 15, 25, 114].
The cellular uptake, localization, biodistribution, circulation and pharmacokinetics of the uncapped AuNPs rely strongly on size and shape [49]. Although these effects are applicable to all AuNPs, the biological effects of citrate-capped AuNPs (cAuNPs) are extensively studied and reviewed. Spherical cAuNPs demonstrated selective in vitro anticancer activity that was size and concentration dependent on murine and human cell lines [3, 51]. Different sizes (10, 20 and 30 nm) of cAuNPs showed differential effects in human cervical carcinoma (HeLa), murine fibroblasts (NIH3T3) and murine melanoma (B16F10) cells. The 20 and 30 nm cAuNPs showed a significant cell death in HeLa cells starting at the lowest concentration of 2.2 µg/mL, while the 10-nm NPs was toxic at concentrations ≥ 8.75 µg/mL. The activity of these NPs was negligible in the noncancerous NIH3T3 cells, especially the 10 and 20 nm. The 20 nm reduced viability by ≤ 5% at the highest concentration (35 µg/mL), and ~ 20% for the 10 and 30 nm. The IC50 values for 10, 20 and 30 nm cAuNPs in the Hela cells were 35, 2.2 and 4.4 μg/mL, respectively, while the IC50 values for noncancerous cells were higher than 35 µg/mL [3]. Using a concentration range of 0.002–2 nM, 13 nm cAuNPs induced apoptosis in rabbit articular chondrocytes and no effects were observed for 3 and 45 nm cAuNPs under the same conditions. The 13 nm cAuNPs induced mitochondrial damage and increased reactive oxygen species (ROS); these actions could not be blocked by pre-treatment with a ROS scavenger, the N-acetyl cysteine [51]. Size-dependent effects were also observed in vivo after injecting cAuNPs of various sizes (3, 5, 8, 12, 17, 37, 50 and 100 nm) into mice (8 mg/kg/week) for 4 weeks. The 8, 17, 12 and 37 nm were lethal to the mice and resulted in tissue damage and death after 14 days of treatment; the other sizes were not toxic and the mice survived the experimentation period. On the contrary, the same-size AuNPs at a concentrations up to 0.4 mM were not toxic to HeLa cells after 24 h exposure [118].
The cAuNPs can interact and accumulate nonspecifically within various tissues and organs in the body, especially in the reticuloendothelial system (RES) organs (blood, liver, spleen, lungs) [55, 119]. This was evident in high-fat (HF) diet-induced obese Wistar rats [55] and Sprague–Dawley rats [119] following acute (1 dose for 24 h) [55] and chronic (1 dose; 0.9, 9 and 90 µg/week over 7 week period) [119] exposure to 14 nm cAuNPs, respectively. Majority of the i.v injected cAuNPs were detected in the liver, spleen, pancreas, lungs, kidneys [55, 119] including the skeleton and carcass of the rats [119]. Chen et al. observed that after intraperitoneal (i.p) injection of a single dose (7.85 µg/g bodyweight) of 21 nm cAuNPs in lean C57BL/6 mice, they accumulated in the abdominal fat tissues and liver after 24–72 h [29], as well as the spleen, kidney, brain and heart in the HF-induced obese mice that were injected with the same dose daily for 9 weeks [50]. The cAuNPs reduced the abdominal WATs (retroperitoneal and mesenteric) mass and blood glucose levels 72 h post-injection [29]. In the diet-induced obese mice, the 21 nm cAuNPs demonstrated anti-inflammatory and anti-obesity effects [50]. They also improved glucose tolerance, enhanced the expression of inflammatory and metabolic markers in the retroperitoneal WATs and liver [50]. Both the 14 and 21 nm cAuNPs showed no sign of toxicity or changes in the markers associated with kidney and liver damage [29, 55, 119].
Similar findings were reported for plant-mediated AuNPs, without targeting molecules they can access, ablate tumors [40, 120] and obese WATs [121] in rodents. Differential uptake, distribution and activity of biogenic AuNPs also vary depending on the size and shape of the NPs. While certain sizes can pass through the vascular network and be retained at the site of the disease; others can be easily filtered out of the system through the RES organs and the mononuclear phagocytic system as shown in Fig. 6 [15, 114]. NPs can be removed by tissue-resident macrophages (TRMs) before they reach the disease cells. Those that escape the TRMs and do not reach the disease site, especially smaller NPs (≤ 5 nm), are excreted through glomerular filtration in the kidney [25, 114]. Pre-treatment with clodronate liposomes depleted the TRMs in the liver and spleen before exposure to 50, 100 and 200 nm AuNPs. This reduced uptake of the AuNPs by the liver, increased their half-life in the blood as well as their accumulation at the tumor site [122]. However, TRMs are not the only obstacle that the AuNPs that rely on EPR effect for uptake must overcome. EPR effect alone can only ascertain ≤ 1% AuNP uptake [15, 114], and depletion of the TRMs prior to treatment resulted in just ≤ 2% of NPs reaching the target [122]. The success of non-targeted AuNPs depends on their ability to reach and accumulate in the diseased tissues, of which passive targeting through the EPR effect might not be efficient. The NPs also need to circulate longer, escape early clearance, and most importantly show reduced bystander effects [25, 123]. These qualities can increase bioavailability and ensure selectivity and efficacy of the AuNPs. These can further be improved by changing the surface chemistry of the AuNPs as discussed below [15, 124].
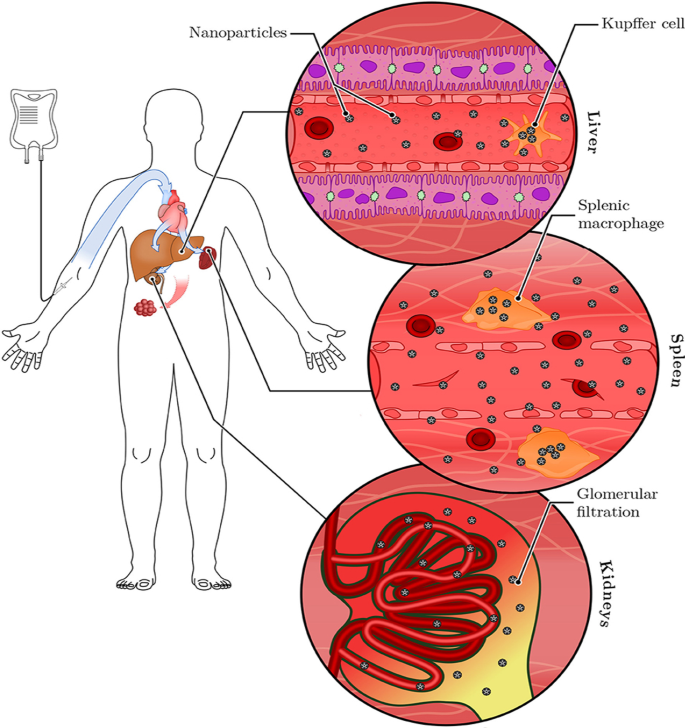
RES-based clearance of systemic administered AuNPs depends on their size. Large AuNPs accumulate in the liver, while smaller AuNPs are likely to end up in the spleen or be excreted in the urine via glomerular filtration. The AuNPs that escape the TRMs could accumulate in the diseased tissues. Reproduced with permission [114]. Copyright 2019, Frontiers in Bioengineering and Biotechnology
Therapeutic Effects of Surface-Functionalized AuNPs
The common strategy in AuNP-based therapeutics involves modifying the AuNP surface with therapeutic agents [3, 124,125,126]. The therapeutic agents can be drugs already used for the treatment of a particular disease or biomolecules with known inhibitory effects on cell signaling. In some instances, the therapeutic AuNPs have also been designed to have molecules that facilitate active targeting of the AuNPs toward specific cells and tissues. The molecules can easily adsorb on the AuNP surface by thiolation, chemical modification using chemistries such as 1-ethyl-3-(3-dimethylaminopropyl) carbodiimide (EDC), streptavidin/biotin binding [3, 124,125,126,127] and ionic interactions based on opposite charges between the NP surface and the biomolecules [124,125,126]. Functionalization of the AuNP surface influences their physicochemical properties and can affect their safety, biocompatibility and mobility. To ensure that the cargo carried by the AuNPs is delivered to the intended site, consideration should thus be given to both the physical and chemical properties of the AuNPs [124,125,126]. It is especially the size, shape, charge and the capping agents of the AuNPs that play an important role in the functionality of the AuNP conjugates [124] and can completely alter the pharmacokinetics of the AuNP-based therapeutics.
Functionalization allows for the development of customized nanosystems to reduce undesirable bystander effects often associated with traditional medicine. Functionalization of AuNPs can also prevent nonspecific adsorption of proteins onto the AuNP surface which can result in the formation a protein corona, resulting in the early clearance of the AuNPs through opsonization by the phagocytic cells [49, 123]. The surface charge of NPs can have a major influence on the behavior of NPs within biological environments. AuNPs with a neutral surface charge are unreactive and have a higher rate of escaping opsonization than charged AuNPs. Hydrophilic NPs will also behave differently to those with hydrophobic surfaces [49, 123]. PEG is one of the polymers most often used to mask AuNPs from phagocytic cells and has been shown to stabilize and enhance the biocompatibility of the AuNPs in numerous in vivo studies [49, 55, 123]. Pegylation improved the biocompatibility of 8.2 nm AuNPs by preventing neutrally and negatively charged AuNPs to bind to cell membranes or localize to any cellular components in African green monkey kidney (COS-1) cells [49]. And when the pegylated AuNPs were functionalized with a polyarginine cell penetrating moiety, the AuNPs were visualized on the cell membrane and inside the COS-1 cells [49]. Cell-penetrating peptides such as nuclear localization signal from SV40 virus, Tat from HIV and polyarginine peptides have been explored in translocation of AuNPs inside all cell type, normal or diseased. However, high specificity is required for clinical applications and can be achieved by taking advantage of the physiological differences between malignant and normal cells. This has been achieved by functionalizing the AuNPs with targeting molecules that recognize cell-specific receptors that are exclusively or overexpressed on the surface of target cells. This way, the AuNPs can be directed and delivered only to cells that express the target receptor. Therefore, conjugation of targeting moieties to the AuNPs (active targeting) will provide more selectivity, reduced bystander toxicity and enhanced efficacy since the AuNPs will be confined only to malignant tissues that express the target receptors [49, 55, 57, 113, 126, 127].
A good example to demonstrate the versatility of AuNPs is shown in Fig. 7, where four different molecules were conjugated onto the AuNPs to target two independent markers and mechanisms [11]. The multifunctional AuNPs were used for the treatment of leukemia (K562DR) cells that are resistant to doxorubicin (Dox). The 40 nm AuNPs were modified with two targeting moieties (folate and AS1411 aptamer) and two therapeutic agents (Dox and anti-miRNA molecules/anti-221). Folate molecule and AS1411 aptamer, respectively, recognize the folate and NCL receptors that are overexpressed on the cell surface and through receptor-mediated endocytosis will traffic the AuNP-conjugate into the cells. The AS1411 aptamer had dual functions, by also targeting the NCL receptor that is expressed inside the cells. After the AuNP-conjugate has been shuttled into the cells, the cargo (AS1411 aptamer, anti-221 and Dox) is off-loaded which independently act on three mechanisms that will synergistically bring about the demise of the cells. AS1411 aptamer together with anti-221 prevented leukemogenesis by suppressing the endogenous NCL and miR-221 function in the NCL/miR-221 pathway, thereby sensitizing the cells to the effects of Dox [11].
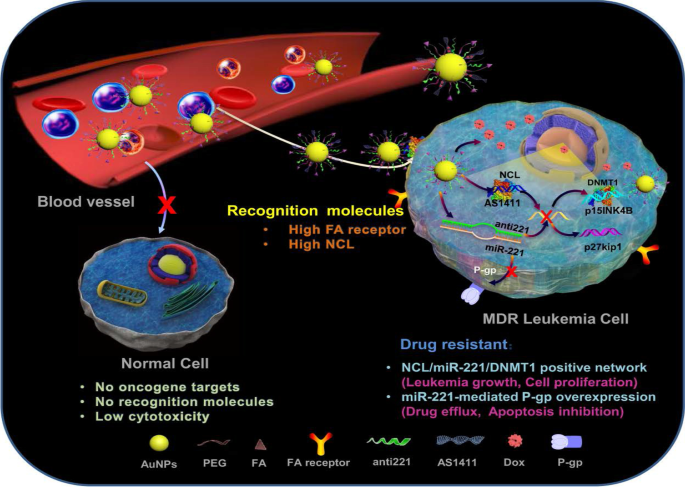
Multifunctional AuNPs in the treatment of multidrug-resistant (MDR) leukemia cells by increasing the sensitivity of the cells to Dox. Reproduced with permission [11]. Copyright 2019. Springer Nature. Folate (FA) receptor
Interestingly, similar dual targeting and treatment effects were achieved with green synthesized AuNPs without any additional molecules. With natural products acting as reducing agents, the biogenic AuNPs might also be more biocompatible than the chemically synthesized NPs [12, 40, 41, 43, 120]. MGF-AuNPs selectively targeted the laminin receptors in prostate (PC-3) and triple-negative breast cancer (MDA-MB-231) cells, and their xenografts in severe combined immunodeficiency (SCID) mice bearing these tumors [12, 40, 120]. In the normal SCID mice, the majority (85% at 30 min increasing to 95% after 24 h) of the i.v-injected MGF-AuNPs accumulated in the liver. Less than 10% were detected in the blood (2.7%), spleen (5%), lungs (0.6%), stomach, intestines and kidneys. When intra-tumorally injected in SCID mice-bearing prostate tumors, only 11% of the MGF-AuNPs were detected in the liver 24 h post-injection, while ~ 80% was in the tumor. Negligible amounts were found in the stomach, carcass and the small intestines. Some of the AuNPs were excreted through the renal and hepatic pathways in the urine and feces after 24 h [40, 120]. Nano Swarna Bhasma, a mixture consisting of AuNPs synthesized from mango peel extracts and phytochemicals from mango, turmeric, gooseberry and gum arabic, showed reduced toxicity toward normal endothelial cells after 48 h compared to the MDA-MB-231 cells [12].
Several studies have demonstrated that AuNPs have potential for clinical application. In combination with conventional drugs, it can be used to sensitize diseased cells to the drug effects [12, 128] and also prevent or reduce drug-related bystander effects [12]. AuNPs improved the pharmacokinetics of chemotherapeutic drugs, such as Dox [43, 129] and 5-fluorouracil (5-FU) [128]. Great improvements were mostly seen in the permeability and retention of drugs in the diseased cells, resulting in enhanced efficacy [130]. Dox-loaded AuNPs, which were non-toxic toward normal mouse fibroblast (L929) cells, also demonstrated selective toxicity toward fibrosarcoma tumors in mice [129]. 5-FU conjugated to the cAuNPs had better activity than 5-FU on its own in colorectal cancer cells [128]. AuNP co-treatment with chemotherapeutic drugs was highly efficient in improving the efficacy of chemotherapeutic drugs [12, 43, 128, 129, 131]. Orally ingested Nano Swarna Bhasma in combination with Dox and Cyclophosphamide reduced tumor volumes in SCID mice-bearing breast tumor cells and also showed acceptable safety profile and reduced bystander effects of the chemotherapeutic drugs in stage IIIA/B metastatic breast cancer patients [12]. Active targeting alone can ensure that the AuNPs are directly delivered into the desired targets, achieving a balance between efficacy and toxicity while minimizing damage to healthy tissues [14, 15, 49]. Controlled drug release is also among the many advantages offered by the AuNP-based systems and is crucial as it allows for localized and selective toxicity [49]. The AuNPs can be designed in such a way that their conjugates respond to internal (glutathione displacement, enzyme cleavable linkers, pH) or external (light, heat) stimuli to function [24, 25, 34, 128].
AuNPs as Transfection Agents in Gene Therapy
The use of AuNPs in gene therapy has shown promising outcomes by facilitating the delivery of genetic material to cells to silence or enhance expression of specific genes [24, 32, 132]. Thus, AuNPs can be used as transfection reagents in gene therapy for the treatment of cancer and other genetic disorders. AuNP conjugates have demonstrated higher transfection efficiency than experimental viral and non-viral gene-delivery vectors including polycationic reagents that has been approved for clinical use [24].
AuNPs are highly conductive and well suited for use as microelectrodes during electroporation for intracellular delivery of biomolecules for disease treatment. AuNPs significantly enhanced the performance of electroporation systems and have been used successfully for the delivery of DNA into hard-to-transfect cells such as the K562 cells [133]. To prevent cell loss which is often associated with electroporation, targeting moieties can be conjugated to the AuNPs to facilitate cellular uptake of AuNP conjugates through receptor-mediated mechanisms [133]. The use of AuNPs to transfect cells with oligonucleotide molecules also has the added advantage of increasing the half-life of these biomolecules and their efficacy [24, 32].
Untargeted AuNP conjugates are passively transported into cells and rely on the surface charge and AuNP shape for efficient transfection [24, 36, 134, 135]. The charge of the biomolecules that are conjugated onto AuNP surface plays a crucial role in their transfection efficiency; for instance, AuNPs functionalized with cationic molecules produce higher transfection efficiency than AuNPs functionalized with anionic molecules. Positively charged amino acids (lysine) can be attached on the NP surface to increase the rate of transfection. AuNSs [24] and AuNRs [36, 134, 135] are commonly used for transfections, and relative to the conventional transfection reagents (X-tremeGENE and siPORT), they inhibited the expression of target gene by > 70% in vitro [134] and in vivo [135]. In these studies, transfection efficiency was quantified based on target expression using RT-PCR and immunostaining [134, 135]. As transfection reagents, AuNPs provide long-lasting effects, localized gene delivery and higher efficacy [36, 134, 135]. Other types of nanomaterials (e.g., polymeric, liposomes, ceramic and carbon nanotubes) had received more attention for use in gene therapy than AuNPs. Six clinical trials using either polymeric or lipid-based nanomaterials for delivery of siRNA in solid tumors have been completed [36, 134, 136]. All of which suffer from low loading efficiency, low stability, and insufficient payload release [36, 136]. On the other hand, transfection systems based on AuNPs make use of easy chemistry that ensures efficient loading capacity and formation of stable complexes [36, 135]. Their safety can be controlled by manipulating their shape, size distribution and surface composition [36].
Antimicrobial Effects of AuNPs
MDR microbes are a major health concern and a leading cause of mortality, worldwide [21, 137,138,139,140,141]. These microorganisms have become resistant to conventional antimicrobial agents, due to over-prescription and misuse of these drugs [142]. No new antibiotics have been produced in over 40 years, mainly because the big pharmaceutical companies have retreated from their antibiotic research programs due to the lack of incentives [143]. As such, new and effective antimicrobial agents are urgently required to combat what could be the next pandemic, the antimicrobial resistance, and avoid surge in drug-resistant infections.
AuNPs are among the new generation of antimicrobial agents under review. They have shown broad antimicrobial (bactericidal, fungicidal and virucidal) effects against a number of pathogenic and MDR microorganisms and thus have potential to overcome microbial drug resistance [21, 142, 144]. Their antimicrobial effects are dependent on their physicochemical properties, especially their size, surface composition, charge and shape [21, 144]. Due to their small size, AuNPs can easily pass through the bacterial cell membrane, disrupt their physiological functions and induce cell death [35]. The exact antimicrobial mechanisms of AuNPs are not yet fully elucidated; despite this, some of the reported modes of actions that results from the interaction of various nanostructured materials (NSMs) with the bacterial cells are illustrated in Fig. 8. The highlighted mechanisms are also implicated in antimicrobial activity of AuNPs, they include induction of microbial death through membrane damage, generation of ROS and oxidative stress, organelle dysfunction, and alteration of gene expression and cell signaling [141].
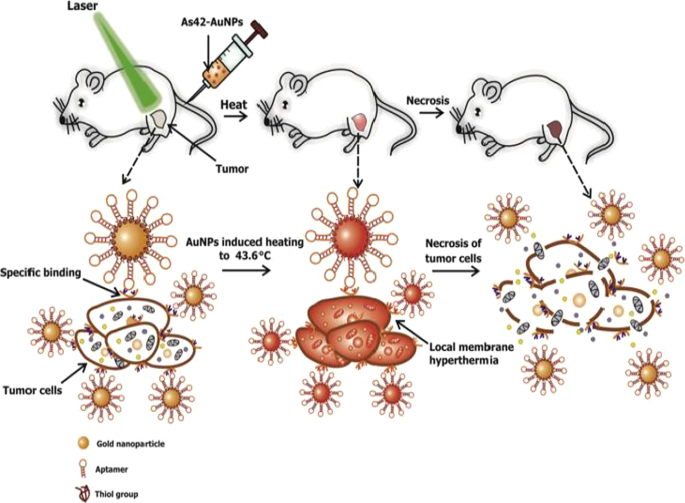
Antimicrobial mode of actions of the NSMs. Various NSMs can induce cell death by altering various biological functions, X represents alteration of cell signaling by de-phosphorylation of tyrosine residues in proteins as one of the mechanisms. Reproduced with permission [141]. Copyright 2018, Frontiers in Microbiology
AuNPs have multiple roles to play toward the development of antimicrobial agents, aside from being antimicrobial agents by themselves; they can serve as drug sensitizers and drug delivery vehicles [35, 58, 132, 145]. These features are applicable to both the chemical and green synthesized AuNPs, which have been reported to have antimicrobial effects against a number of human [21, 145,146,147] and waterborne [148] pathogenic strains. Generally, the test bacteria had shown low susceptibility toward the chemically synthesized AuNPs, i.e., the cAuNPs [21, 146, 147] and the NaBH4 -reduced AuNPs [149]. This was due to the repulsive forces between the negative charges on the AuNP surfaces and bacterial cells, thus preventing the interaction between AuNPs and the bacteria [21]. The activity of chemically synthesized AuNPs is based on their size, shape, concentration and exposure time. As an example, one study reported that NaBH4 -reduced AuNPs had no activity against Staphylococcus aureus (S. aureus ) and Escherichia coli (E. coli ) at 500 µg/mL for the duration of 6 h [149]. In contrast, another study showed a significant dose (1.35, 2.03 and 2.7 μg/mL) and size (6–34 nm vs 20–40 nm) dependent antibacterial effects of the NaBH4 -reduced AuNPs on Klebsiella pneumonia , E. coli , S. aureus and Bacillus subtilis [145].
The AuNPs are either used alone or in combination with other antimicrobial agents to treat microbial infections [35, 58, 132, 145]. When used in combination with other antimicrobial agents, the AuNP conjugates resulted in synergistic antimicrobial effects that surpassed the individual effects of the AuNPs and drugs [21, 35, 58, 132, 150]. These drugs were conjugated onto the AuNPs by either chemical methods [4, 151] or the drugs were used as reducing and capping agents [21, 149]. By so doing, the AuNPs improved drug delivery, uptake, sensitivity and efficacy. Some of the FDA-approved antibiotics and non-antibiotic drugs that were loaded onto the AuNPs are shown in Table 1 [4, 21, 149, 152]. Ciprofloxacin [152], cefaclor [149], lincomycin [4], kanamycin [21], vancomycin, ampicillin [151] and rifampicin [32] are among the antibiotics loaded on the AuNPs and demonstrated the versatility of AuNPs. These strategies were successful with various sizes and shapes of AuNPs, including gold silica nanoshells [152], AuNP-assembled rosette nanotubes [151] and AuNPs encapsulated in multi-block copolymers [153]. For instance, cefaclor-reduced AuNSs inhibited the growth of S. aureus and E. coli within 2–6 h depending on the concentration (10–50 µg/mL), while complete bacterial growth inhibition by the drug alone was only observed at 50 µg/mL after 6 h. The minimum inhibitory concentration (MIC) of the treatments was 10 µg/mL and 50 µg/mL for cefaclor-AuNPs and cefaclor, respectively [149].
AuNPs have presented properties that make them ideal candidates as alternative antimicrobial agents; the most important being their broad antimicrobial activity [21, 35, 58, 132, 150]. Owing to their biocompatibility and easily modifiable surface, microorganisms are less prone to developing resistance toward AuNPs [21]. For example, the kanamycin (Kan)-resistant bacteria (S . bovis , S . epidermidis , E . aerogenes , P . aeruginosa and Y . pestis ) showed increased susceptibility toward Kan-reduced AuNPs. The MIC values for Kan-AuNPs on the test bacteria were significantly reduced to < 10 µg/mL when compared to the MIC values for Kan alone at 50–512 µg/mL. This shows that AuNPs can restore the potency of antibiotics toward the drug-resistant strains by facilitating the uptake and delivery of the antimicrobial agents [21]. AuNPs can enhance drug-loading capacity and control the rate at which the drugs are released. AuNP hybrids with the multi-block copolymers increased the loading capacity of rifampicin and the drug’s half-life to 240 h. By sustaining the drug in the system for that long, ensured slow release of rifampicin from AuNPs at the target sites after oral administration of the AuNP conjugates to rats for 15 days. The drug on the surface was released within 24 h followed by the drug trapped in the polymer matrix after 100 h. And lastly, the drug entrapped between the AuNPs and the polymer matrix took over 240 h to be released in the interstitial space [153].
The AuNP hybrids also allow for the conjugation of multiple molecules with independent but synergistic functions. This was demonstrated by co-functionalization of the AuNPs with antimicrobial peptide (LL37) and the pcDNA that encode for pro-angiogenic factor (vascular endothelial growth factor, VEGF) and used in the treatment of MRSA-infected diabetic wounds in mice [132]. The AuNPs served dual functions, as a vehicle for the biomolecules, and also as transfection agent for the pcDNA. After topical application of the AuNP conjugates on the wound, the LL37 reduced MRSA colonies, while the pcDNA promoted wound healing by inducing angiogenesis through the expression of VEGF [132].
AuNPs have been shown to confer activity and repurpose some non-antibiotic drugs toward antimicrobial activity. The examples of repurposed drugs, which were used for the treatment of diseases other than bacterial infections, include 5FU [58], metformin [147] and 4,6-diamino-2-pyrimidinethiol (DAPT) [13, 112]. AuNPs as drug carriers are able to transport the drugs into the cells and allow direct contact with cellular organelles that resulted in their death [58, 147]. 5FU is an anti-leukemic drug, when attached to AuNPs was shown to kill some bacterial (Micrococcus luteus , S. aureus , P. aeruginosa , E. coli ) and fungal (Aspergillus fumigatus , Aspergillus niger ) strains [58]. While bacteria are resistant to DAPT, DAPT-AuNPs displayed differential antibacterial activity against the Gram-negative bacteria. Furthermore, conjugation of non-antibiotic drugs (e.g., guanidine, metformin, 1-(3-chlorophenyl)biguanide, chloroquine diphosphate, acetylcholine chloride, and melamine) as co-ligands with DAPT on AuNPs exerted non-selective antibacterial activity and a two–fourfold increased activity against Gram-negative bacteria [13]. When used in vivo, orally ingested DAPT-AuNPs showed better protection by increasing the intestinal microflora in E. coli -infected mice. After 4 weeks of treatment, the DAPT-AuNPs cleared the E. coli infection with no sign of mitochondrial damage, inflammation (increase in firmicutes ) or metabolic disorders (reduction in bacteroidetes ) in the mice [112].
The virucidal effects of the AuNP-based systems have been reported against several infectious diseases caused by influenza, measles [154], dengue [155, 156] and human immunodeficiency [115] viruses. Their anti-viral activity was attributed to the ability of AuNPs to either deliver anti-viral agents, or the ability to transform inactive molecules into virucidal agents [154, 156]. AuNPs synthesized using garlic water extracts inhibited measles viral growth in Vero cells infected with the measles virus. When the cells were exposed to both the virus and AuNPs at the same time, they blocked infection of Vero cells by the measles virus [154]. The AuNPs were nontoxic to the Vero cells up to a concentration of 100 µg/mL but inhibited viral uptake by 50% within 15–30 min at a concentration of 8.8 μg/mL [154]. Based on the Plaque Formation Unit assay, the viral load was reduced by 92% after 6 h exposure to 8.8 μg/mL of the AuNPs. The AuNPs interacted with the virus directly and blocked its transmission into the cells [154]. Modification of the AuNP surface with ligands that bind to the virus [156] or anti-viral agents [115, 155] protected them from degradation, enhanced their uptake and delivery onto the cells. The charge of the AuNPs also played a role, with cationic AuNPs being more effective in the delivery and efficacy of the AuNPs than the anionic and neutrally charged NPs. Cationic AuNPs complexed with siRNA inhibited dengue virus-2 replication in dengue virus-2-infected Vero and HepG-2 cells and also the virus infection following pre-treatment of the virus with AuNPs [155]. Inactive molecules are transformed into highly potent anti-viral agents after conjugation to AuNPs. One such example is the transformation of SDC-1721 peptide, a derivative of TAK-779, which is an antagonist of CCR5 and CXCR3 receptors for HIV-1 strain. SDC-1721 has no activity against the HIV-1, but when conjugated to the AuNPs it inhibited HIV-1 infection of the human phytohemagglutinin-stimulated peripheral blood mononuclear cells. The inhibitory effects of SDC-1721-AuNPs were comparable to the TAK-779 [115].
AuNPs as PT Agents
Diseased cells are sensitive to temperatures above 40 °C; cancer cells in particular appear to be even more sensitive to these high temperatures. Studies have shown that high fevers in cancer patients either reduced the symptoms of cancer or completely eradicated the tumors as a result of erysipelas infections [33, 157, 158]. Historically, fevers induced by bacterial infections, hot desert sand bath, or hot baths were used to increase the body temperature in order to kill the cancer cells [157]. These findings gave birth to PT therapy (PTT), which is mostly used for the treatment of cancer. PTT makes use of organic photosensitizers (indocyanine green, phthalocyanine, heptamethine cyanine) that are irradiated by the external source to generate heat energy that will increase the temperature to 40–45 °C (hyperthermia) in the target cells. Hyperthermia then triggers a chain of events (such as cell lysis, denaturation of the genetic materials and proteins), resulting in the destruction of the diseased cells [57, 158,159,160].
The organic dyes are used alone, or in combination with chemotherapy and radiotherapy for enhanced efficacy [157, 160]. Ideally, the effects of the PT agents must be confined to target cells and display minimal bystander effects. However, the organic PT dyes have several limitations such as toxic bystander effects, susceptibility to photobleaching and biodegradation [159]. In recent years, AuNPs are being explored as alternative PT agents as they exhibit strong plasmonic PT properties, and depending on their shape, they can absorb visible or NIR light. Absorption of light in the NIR spectrum is an added advantage that can allow deep tissue PTT [158, 161, 162]. Unlike organic dyes, AuNPs operate in an optical window where the absorption of light by interfering biological PT agents such as hemoglobin, melanin, cytochromes and water is very low [158, 161, 162].
The practicality of AuNP-based PTT has been demonstrated through in vitro and in vivo studies [158, 162, 163]. When the AuNPs are exposed to light, they can convert the absorbed light energy into thermal energy within picoseconds [57, 158, 159], consequently activating cell death via necrosis or apoptosis in the target cells or tissues. AuNP-based hyperthermia in diseased cells has been reported to occur at half the amount of the energy required to kill normal cells, thus perceived to be safer and better PT agents than the conventional dyes [33, 160]. AuNPs can be easily modified to have localized and enhanced PT activity by targeting and accumulating in only diseased cells through either active or passive targeting. And since the tumor environment is already hypoxic, acidic, nutrient starved and have leaky vasculature, the tumors will be most sensitive to the AuNP-based hyperthermia than the surrounding healthy cells and tissues [33, 160].
AuNP-based PTT has been extensively studied [158, 161, 162] and established that AuNPs (e.g., AuNRs, nanocages and nanoshells) that absorb light in the NIR spectrum are best for in vivo and deep tissue PTT [161]. While the ones that absorb and emit light in the visible spectrum (AuNSs and hollow AuNPs) have been demonstrated to treat diseases that affect shallow tissues (up to a depth of 1 mm), which could be of benefit to superficial tumors [158, 161, 162], ocular surgery [164, 165], focal therapy and vocal cord surgery [158, 165]. Although the PTT effects of AuNSs are limited in vivo or for use in deep tissues, combination therapy or active targeting can be incorporated to facilitate target-specific effects [158, 161, 163]. The AuNPs in the combination therapy will serve dual functions as both drug sensitizer and a PT agent, and was shown to enhance anticancer effects of chemotherapeutic drugs [158, 162, 163]. AuNS-Dox combination demonstrated enhanced cancer cell death after laser exposure when compared to the individual effects of the AuNSs and Dox with and without laser treatment [158].
Active targeting on its own can also improve AuNP uptake, localization and target-specific PT effects, which can be viewed in real time by adding fluorophores. AuNSs (25 nm) loaded with transferrin targeting molecules and FITC were shown to accumulate and destroy human breast cancer cells at a higher rate than in non-cancer cells and had better efficacy than the untargeted AuNSs [57]. An independent study also demonstrated that DNA aptamers (As42)-loaded AuNSs (As42-AuNP) induced selective necrosis in Ehrlich carcinoma cells that express HSPA8 protein, a receptor for the aptamers. None of these effects were observed in blood and liver cells mixed with target cells, or cells treated with the AuNSs without laser treatment [163]. The PT effects of the As42-AuNP were replicated in mice transplanted with Ehrlich carcinoma cells in their right leg. As shown in Fig. 9, tail-vein injections of As42-AuNPs followed by laser irradiation resulted in targeted PT destruction of the cancer cells. The As42-AuNPs reduced tumor size in a time-dependent manner; cell death was attributed to increased temperature up to 46 °C at the tumor site. The tumor in mice treated with As42-AuNPs without laser treatment and the AuNPs conjugated with nonspecific DNA oligonucleotide continued to grow but at the lower rate compared to mice injected with PBS. This suggests that the AuNPs were also localized in the tumor [163]. In cases where AuNSs are not efficient for deep tissue PTT, other shapes such as nanocages, nanoshells and AuNRs can be used [158]. Alternately, the visible light absorption of the AuNSs can be shifted to NIR by using processes such as two-photon excitation [57].
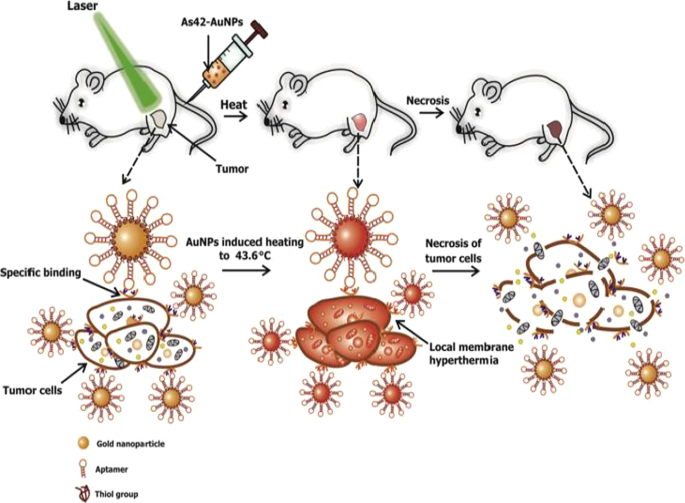
In vivo plasmonic PT therapy of cancer cells using targeted AuNSs. As42-AuNPs localized in HSPA8-expressing tumor cells after i.v injection. Exposure to laser treatment resulted in hyperthermia that caused cancer cell death. Reproduced with permission [163]. Copyright 2017, Elsevier
The PT effects of the AuNPs have also been reported for the reversal of obesity [52, 56], using hollow AuNSs (HAuNSs) [52] and AuNRs [56] for the PT lipolysis of the subcutaneous white adipose tissue (sWAT) in obese animals. The HAuNSs were modified with hyaluronate and adipocyte targeting peptide (ATP) to produce HA–HAuNS–ATP conjugate [52]. Hyaluronate was used to ensure topical entry of the HA–HAuNS–ATP through the skin [52, 166], while ATP will recognize and bind to prohibitin once the HAuNSs are internalized. Prohibitin is a receptor that is differentially expressed by the endothelial cells found in the WAT vasculature of obese subjects [5, 52, 55]. The HA–HAuNS–ATP was topically applied in the abdominal region of the obese mice, and through hyaluronate were transdermally shuttled through the epidermis into the dermis where the ATP located the sWATs (Fig. 10) . Illumination of the target site with the NIR laser selectively induced PT lipolysis of the sWAT in the obese mice and reduced their body weight [52]. The AuNRs were used in the photothermolysis-assisted liposuction of the sWATs in Yucatan mini pigs. The untargeted PEG-coated AuNRs (termed NanoLipo) were injected in the sWATs through an incision, followed by laser illumination to heat up the sWATs, which was then aspirated using liposuction. The amount of fat removed from NanoLipo-treated porcine was more than the one removed with conventional suction-assisted lipectomy (SAL). NanoLipo-assisted fat removal had several advantages over the conventional SAL; it took less time (4 min) for liposuction compared to 10 min for SAL, the swelling in the treated site healed faster, and the weight loss effects lasted over 3 months post-liposuction [56].
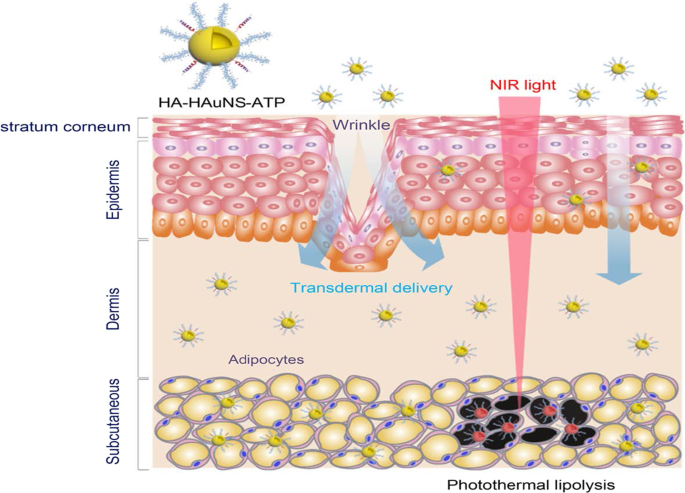
PT lipolysis of the sWATs using HA-HAuNS-ATP. The ATP was conjugated to the AuNSs for targeted delivery and destruction of the prohibitin-expressing sWATs after NIR laser exposure. Reproduced with permission [52]. Copyright 2017, American Chemical Society
AuNP-based PTT clearly offers a lot of advantages compared to the conventional agents. Their biocompatibility allows for broader applications both in vitro and in vivo. Moreover, they can be customized based on their shapes for shallow (AuNSs) [158, 161, 162] or deep tissue (AuNRs and stars) PTT [158, 161]. At 1–100 nm diameter, AuNPs and its conjugates can circulate long enough to reach and accumulate in the target tissues, with or without targeting moieties [159, 167]. Active targeting can be used to ensure localized PT effects through various routes of administration and might be effective for solid and systemic diseases. AuNP-based PTT can also be used to sensitize cancer cells when administered in combination with chemotherapy, gene therapy and immunotherapy [159]. Therefore, AuNP-based PTT has potential for treatment of chronic diseases [161].
Toxicity of AuNPs
AuNPs can play an important role in medicine, as demonstrated by the preclinical and clinical studies under review. Their full potential in clinical application as both diagnostic and therapeutic agents can only be realized if they do not pose any health and environmental hazards. While their use in vitro appears to be inconsequential, in vivo application can be hampered by their potential toxicity, which could be detrimental to human health. A major concern with their clinical use is that AuNPs are non-biodegradable and their fate in biological systems has not been fully studied [5, 30]. Although AuNPs are considered to be bio-inert and compatible, their properties (size, shape, charge and composition) raise concerns as they can alter their pharmacokinetics when used in biological environment [27, 34, 118]. The toxicity of AuNPs of varying sizes and shapes has been demonstrated in animals [27, 118]. These NPs can accumulate in the RES organs where they induce damage.
AuNPs are 1–100 nm in diameter which makes them smaller than most of the cellular components. At these sizes, AuNPs can passively transverse cellular barriers and blood vessels by taking advantage of the EPR effect in pathological cells. AuNPs with smaller diameters (1–2 nm) can easily penetrate cell membranes and biologically important cellular organelles such as mitochondria and nuclei [7, 168]. Accumulation of AuNPs in these organelles induces irreversible damage that can cause cellular demise. On the contrary, AuNPs larger than 15 nm are restricted to the cytoplasmic spaces and unable to penetrate internal organelles [168]. These features are desirable for targeting pathological cells, however, AuNPs can also be taken up by healthy cells and alter their physiology [118]. Administration of AuNP-based therapeutics can be done via different routes (i.e., intranasal, oral, transdermal, i.p or i.v) and transported through blood vessels into different tissues and organs [34, 118]. They are able to pass through the blood brain barrier and the placental barrier [34]. Toxicity is size dependent, with certain sizes of AuNPs being well tolerated, while others could be lethal to healthy tissues. Unfunctionalized AuNSs at 8, 17, 12, 37 nm caused physical changes (i.e., change the fur color, loss of bodyweight, camel-like back and crooked spine) within 14 days of treatment (2 doses of 8 mg/kg/week) in rats [118]. Most (> 50%) of the rats died within 21 days (i.e., after 3 doses), and abnormalities in the RES organs (liver, lungs and spleen) were observed. On the contrary, mice treated with 3, 5, 50 and 100 nm AuNPs were not affected by the NPs and no adverse effects or death occurred throughout the duration (50 days) of the study [118]. In diet-induced obese rats that received i.v injections of 14 nm cAuNPs, the NPs were detected in various tissues after 24 h and were mostly confined to the RES organs [55].
The shape, charge and surface chemistry of AuNPs can influence their toxicity. These factors can determine how AuNPs will interact with the biological systems, their cellular uptake and effects on the cells. AuNSs are readily taken up by cells and proven to be less toxic than other shapes such as rods and stars. AuNP surfaces are charged and will influence how they interact and behave within a biological environment [169]. Cationic AuNPs are likely to be more toxic compared to neutral and anionic AuNPs, as their charge allows these NPs to easily interact with negatively charged cell membranes and biomolecules such as DNA. Both the positively and negatively charged AuNPs have been associated with mitochondrial stress, which was not observed with the neutrally charged AuNPs [34, 35].
The shell that forms on the surface of the AuNP core can also influence the functioning of the NPs. These are usually reducing and/ or stabilizing agents such as citrate and CTAB, and once subjected to a biological environment, these molecules can cause either the desorption or absorption of biomolecules found in the biological environment. This can result in the formation of a corona or cause the NPs to become unstable. Citrate- and CTAB-capped AuNPs are highly reactive, which can facilitate the attachment of biocompatible polymers such as PEG, polyvinyl-pyrrolidone, poly (acrylic acid), poly(allylamine hydrochloride), and polyvinyl-alcohol) or biomolecules such as albumin and glutathione to prevent the formation of AuNP-corona with serum proteins. These molecules serve as a stabilizing agent and form a protective layer that can mask the AuNPs from attacks by phagocytes [7, 29, 34, 170] and prevent off-target toxicity [7]. As discussed in “AuNP-Based Therapies” section, AuNPs can be functionalized with targeting and therapeutic agents to define their targets and effects [34].
In addition to their physicochemical properties, the dosage, exposure time and environmental settings also influence the activity of AuNPs. Lower doses and short-term exposure times might render AuNP as nontoxic, while increasing these parameters will lead to cytotoxic effects [34]. Moreover, in vitro studies do not always simulate in vivo studies. At times, AuNPs that seem to be nontoxic in cell culture-based experiments end up being toxic in animal experiments. Many factors could be responsible for these discrepancies [118], and some steps have been identified that can guarantee the safety of AuNPs in biomedical applications. The biocompatibility and target specificity of AuNPs can be improved by modifying the surface of the NPs. Attaching targeting moieties on the AuNPs can channel and restrict their effects to specific targets or pathological cells [5, 55, 127]. Modification of AuNP surface with bio-active peptides provides a platform for developing multifunctional AuNPs with enhanced specificity, efficacy and potentially sustainable effects [11, 127]. All of these effects will be instrumental in the design and development of AuNP-based systems for clinical applications.
Clinical Application of AuNPs
Nanotechnology has the potential to shape the future of healthcare systems and their outcomes. Its promise of creating highly sensitive and effective nanosystems for medicine has been realized with the introduction of organic nanoformulations for cancer treatment. These systems have already paved the way for nanomaterials into clinical applications:doxil and abraxane have been in the market for over two decades and demonstrated the potential of nanotechnology in medicine [1, 2]. More recently, this technology has been used for the development of the SARS-CoV-2 lipid NP-based vaccine to fight against the COVID-19 pandemic [171]. Inorganic nanosystems such as AuNPs offer many advantages over their organic counterparts, yet few of these systems are used clinically (Table 2) [19, 32].
While several AuNP-based drugs are some of the inorganic nanomaterial-based drugs that were tested in clinical trials, they are not progressing at the same rate as organic liposome-based nanodrugs. Aurimune (CYT-6091) and aurolase were the first of AuNP-based formulations to undergo human clinical trials for the treatment of solid tumors. CYT-6091 clinical trials started in 2005 for delivery of recombinant TNF-α as an anticancer therapy in late-stage pancreatic, breast, colon, melanoma, sarcoma and lung cancer patients. CYT-6091 consists of 27-nm cAuNPs loaded with TNF-α and thiolated PEG. The CYT-6091 nanodrug has achieved safety and targeted biologic response at the tumor site at a dose lower than that required for TNF-α alone [16, 17]. CYT-6091 is approved and yet to start phase II clinical trials in combination with chemotherapy. Based on phase II clinical trial strategy, several variants of CYT-6091 have been developed and tested in preclinical studies. All the nanosystems contain TNF-α with either chemotherapy (paclitaxel, dox and gemcitabine), immunotherapy (Interferon gamma) or apoptosis inducing agents attached to the 27 nm cAuNPs [14,15,16]. The AuNP conjugates preferentially accumulated in the tumor sites after systemic administration through the EPR effect and vascular targeting effects of the TNF-α. The AuNPs were not detected in the healthy tissues, and the anti-tumor effects of TNF-α were restricted to the tumor environment [14, 16, 19].
The first clinical trial for the PT treatment with AuroLase® for refractory and/or recurrent head and neck cancers was completed. Information on the outcome of this trial is still pending. The second trial is set to evaluate the effects of AuroLase® on primary and/or metastatic lung tumors in patients where the airway is obstructed [19]. The number of human trials based on AuNP-based formulation is increasing, covering the treatment of a wide range of medical conditions including skin, oral, heart and neurological diseases. AuNP-formulation (150 nm silica-gold nanoshells coated with PEG), which is similar to AuroLase®, was approved for PT treatment of moderate-to-severe inflammatory acne vulgaris. The nanoshells were topically applied on the acne area and transdermally delivered into the follicles and sebaceous ducts through low-frequency ultrasound or massage. Nanoshells applied through massage were effective in penetrating the shallow skin infundibulum (90%) and the sebaceous gland (20%), while the low-frequency ultrasound can penetrate both shallow and deep skin tissues. NIR laser treatment resulted in focal thermolysis of the sebaceous glands in the affected area and disappearance of the acne [18, 167]. The gold–silica nanoshells were well-tolerated, showed no systemic toxic effects with minor side effects (reddiness and swelling) at the treatment site [18]. AuNPs offer many health benefits based on their unique properties but at the same time have raised a lot of political and ethical issues, and resulted in termination of some clinical studies (NCT01436123).
Conclusion and Future Perspectives
Applications of AuNPs in biomedicine are endorsed by their unique physicochemical properties and have shown great promise as theranostic agents. The increasing interest in biomedical applications of AuNPs is further encouraged by the biocompatibility and medical history of bulk gold, which suggests that the gold core in AuNPs will essentially display similar or improved properties [3]. But at the same time their small size can infer unique properties that will completely change their pharmacokinetics [144]. The diverse biomedical applications of AuNPs in diagnostics and therapeutics herein discussed demonstrate their potential to serve as adjunct theranostic agents. They can be used as drug delivery, PTT, diagnostic and molecular imaging agents [12, 33, 128]. In time, and with better knowledge of mechanisms of action, more AuNP-based systems will obtain approval for clinical use. However, the excitement of these biomedical applications of AuNPs should unequivocally be balanced with testing and validation of their safety in living systems before any clinical applications.
In conclusion, more work needs to be done to taper the toxicity of AuNPs. This can be achieved by introducing biocompatible molecules on their surface [14, 15, 58, 159], and developing new and better synthesis methods, such as the use of green chemistry to produce biogenic NPs. All these developments may further broaden the applications of AuNPs in nanomedicine. AuNPs are non-biodegradable, and off-target distribution could result in chronic and lethal effects. All these concerns must be addressed before clinical translation; the existing trials will soon provide some clarity on their impact in human health. Should their health benefits outweigh their potential risks as is the case with the existing clinical drugs, it is a matter of time before they are approved for clinical use.
Availability of Data and Materials
All the information in this paper was obtained from the studies that are already published and referenced accordingly.
Abbreviations
- 5-FU:
-
5-Fluorouracil
- AD:
-
Alzheimer's disease
- ADDLs:
-
Amyloid-beta-derived diffusible ligands
- ASOs:
-
Antisense oligonucleotides
- AuNPs:
-
Gold nanoparticles
- AuNPsQ:
-
Quantum-sized AuNPs
- AuNRs:
-
Gold nanorods
- AuNSs:
-
Gold nanospheres
- BCA:
-
Bio-barcoding assay
- cAuNPs:
-
Citrate-capped AuNPs
- COVID-19:
-
Corona virus disease 2019
- CSF:
-
Cerebrospinal fluid
- CT:
-
Computed tomography
- CTAB:
-
Cetyltrimethylammonium bromide
- DABCYL:
-
4-((4′-(Dimethyl-amino)-phenyl)-azo)benzoic acid
- DAPT:
-
4,6-Diamino-2-pyrimidinethiol
- Dox:
-
Doxorubicin
- EDC:
-
1-Ethyl-3-(3-dimethylaminopropyl) carbodiimide
- EPR:
-
Enhanced permeability and retention
- FA:
-
Folate
- FDA:
-
Food and Drug Administration
- FITC:
-
Fluorescein isothiocyanate
- FRET:
-
Fluorescence resonance energy transfer
- HAuNSs:
-
Hollow AuNSs
- HF:
-
High-fat
- HU −1 :
-
Hounsfield unit
- LFAs:
-
Lateral flow assays
- LSPR:
-
Localized surface plasmon resonance
- MDR:
-
Multidrug resistant
- MGF:
-
Mangiferin
- MPA:
-
Mercaptopropionic acid
- MWPLP:
-
Microwave-induced plasma-in-liquid process
- NCL:
-
Nucleolin
- NIR:
-
Near-infrared
- NSMs:
-
Nanostructured materials
- P. jirovecii :
-
Pneumocystis jirovecii
- PEG:
-
Polyethylene glycol
- PSA:
-
Prostate-specific antigen
- PSMA:
-
Prostate-specific membrane antigen
- PT:
-
Photothermal
- PTT:
-
Photothermal therapy
- QDs:
-
Quantum dots
- RES:
-
Reticuloendothelial system
- ROS:
-
Reactive oxygen species
- SARS-CoV-2:
-
Severe acute respiratory syndrome-coronavirus-2
- SCID:
-
Severe combined immunodeficiency
- SPR:
-
Surface plasmon resonance
- sWAT:
-
Subcutaneous white adipose tissue
- TOAB:
-
Tetrabutylammonium bromide
- TRMs:
-
Tissue-resident macrophages
- VEGF:
-
Vascular endothelial growth factor
- WAT:
-
White adipose tissue
Nanomatériaux
- Nanoparticules d'or pour capteurs chimio
- Nanoparticules pour le traitement du cancer :progrès actuels et défis
- Avancées et défis des nanomatériaux fluorescents pour la synthèse et les applications biomédicales
- Composites de graphène et polymères pour applications de supercondensateurs :une revue
- La préparation de la nanostructure jaune-enveloppe Au@TiO2 et ses applications pour la dégradation et la détection du bleu de méthylène
- Polyglycérol hyperramifié modifié comme dispersant pour le contrôle de la taille et la stabilisation des nanoparticules d'or dans les hydrocarbures
- Préparation de micromatériaux hybrides MnO2 revêtus de PPy et de leurs performances cycliques améliorées en tant qu'anode pour batteries lithium-ion
- Fabrication, caractérisation et cytotoxicité de nanoparticules de carbonate de calcium dérivées de coquilles d'or conjuguées de forme sphérique pour des applications biomédicales
- Progrès récents dans les méthodes de synthèse et les applications des nanostructures d'argent