Avancées et défis des nanomatériaux fluorescents pour la synthèse et les applications biomédicales
Résumé
Avec le développement rapide de la nanotechnologie, de nouveaux types de nanomatériaux fluorescents (FNM) ont vu le jour au cours des deux dernières décennies. L'échelle nanométrique confère aux FNM des propriétés optiques uniques qui jouent un rôle essentiel dans leurs applications en bio-imagerie et détections dépendantes de la fluorescence. Cependant, étant donné que la faible sélectivité ainsi que la faible efficacité de photoluminescence des nanomatériaux fluorescents entravent dans une certaine mesure leurs applications en imagerie et en détection, les scientifiques sont toujours à la recherche de nouveaux FNM avec de meilleures propriétés. Dans cette revue, une variété de nanoparticules fluorescentes sont résumées, notamment les points quantiques semi-conducteurs, les points de carbone, les nanoparticules de carbone, les nanotubes de carbone, les nanomatériaux à base de graphène, les nanoparticules de métaux nobles, les nanoparticules de silice, les phosphores et les cadres organiques. Nous soulignons les avancées récentes des derniers développements dans la synthèse des FNM et leurs applications dans le domaine biomédical ces dernières années. En outre, les principales théories, méthodes et limites de la synthèse et des applications des FNM ont été revues et discutées. De plus, les défis de la synthèse et des applications biomédicales sont également systématiquement résumés. Les orientations et perspectives futures des FNM dans les applications cliniques sont également présentées.
Introduction
Les colorants organiques conventionnels ont rencontré des difficultés dans leur application en biomédecine en raison de leurs défauts inhérents tels que la cytotoxicité et la mauvaise biocompatibilité [1]. Cependant, l'émergence des nanomatériaux fluorescents montre un grand potentiel dans le remplacement des colorants organiques conventionnels. Les scientifiques ont consacré beaucoup de temps et d'efforts à la recherche de nanomatériaux fluorescents, et les réalisations pertinentes en matière de synthèse et d'applications sont plus qu'inspirantes.
La forme, la taille et la structure des nanomatériaux fluorescents déterminent leurs propriétés physiques et chimiques, qui ont une influence considérable sur leurs performances. Par conséquent, la synthèse contrôlable de nanomatériaux fluorescents est devenue un sujet de recherche brûlant. Les conditions expérimentales optimales de synthèse contribuent à la taille, la morphologie et la stabilité les plus appropriées des nanomatériaux fluorescents. Ces dernières années, de nombreux efforts ont été déployés pour améliorer la biocompatibilité des nanomatériaux fluorescents en améliorant les méthodes de synthèse [2]. Les ions métalliques étaient généralement dopés avec des points de carbone (CD) ou des points quantiques (QD) pour fonctionnaliser la surface des nanomatériaux fluorescents dans le passé. Cependant, la fluorescence inefficace et la toxicité sous-jacente constituaient une menace pour leurs applications en bio-imagerie et en biomarquage [3]. Compte tenu de ces problèmes, Zuo et al. ont rapporté un système de livraison de gènes CDs à haute efficacité. Les CD dopées au fluor ont été synthétisées par procédé solvothermique, et des sites de charge positive pour la délivrance de gènes peuvent être fournis par la polyéthylèneimine ramifiée (b-PEI) [4]. On peut s'attendre à ce que les nouvelles méthodes de modification de surface soient un domaine de recherche de pointe à l'avenir.
De nombreux efforts ont été déployés pour explorer le potentiel des nanomatériaux fluorescents pour des applications biomédicales qui incluent la bio-imagerie, la biodétection et certaines méthodes de thérapie, comme le montre la Fig. 1. La fluorescence fiable pour l'application dépend de leurs propriétés physiques et chimiques [5]. Par conséquent, les travaux de recherche sur l'amélioration de leurs propriétés telles que la toxicité, l'hydrophilie et la biocompatibilité ont joué un rôle important dans la réalisation de l'utilisation extensive des nanomatériaux fluorescents dans les domaines biomédicaux. Avec le taux croissant de certaines maladies comme le cancer, il existe une demande croissante de nouvelles stratégies de diagnostic et de thérapie avec une précision et une conformité plus élevées de la part des patients [6]. Actuellement, le dopage par ions métalliques ou non métalliques et la modification de surface des nanomatériaux fluorescents sont toujours les techniques dominantes pour améliorer leur efficacité et leur biocompatibilité PL [7], et les recherches correspondantes ouvrent de nouvelles visions des applications biomédicales des nanomatériaux fluorescents.
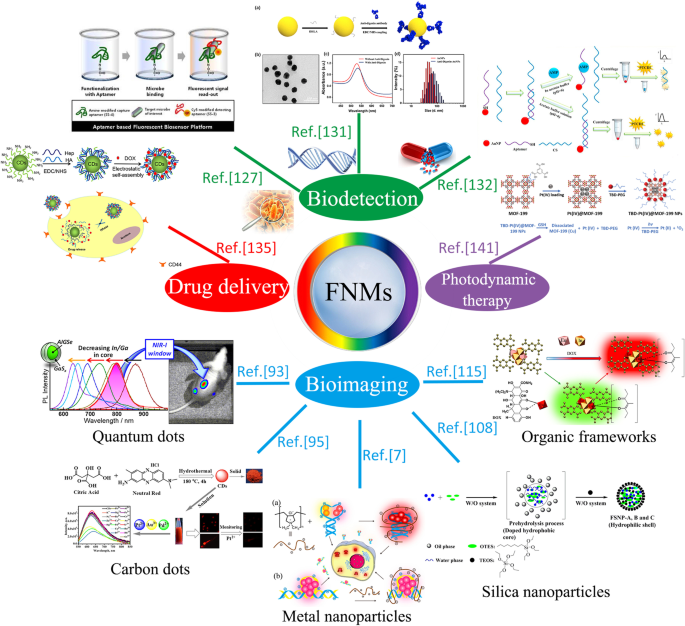
Le schéma de synthèse des applications biomédicales des nanomatériaux fluorescents
Compte tenu du grand potentiel que possèdent les nanomatériaux fluorescents dans le domaine biomédical, cette revue met l'accent sur les dernières avancées et améliorations. Les scientifiques se sont consacrés à la fonctionnalisation de la surface des nanomatériaux fluorescents et à leurs performances dans des applications biomédicales. Ce n'est qu'avec des stratégies de synthèse conçues de manière raisonnable que les matériaux fluorescents peuvent être dotés de la qualité d'une efficacité PL élevée et d'une bonne biocompatibilité, qui sont vitales pour leurs applications dans le domaine biomédical. Nous espérons que cette revue sur la synthèse et les applications des matériaux fluorescents pourra aider les lecteurs à comprendre la tendance générale du développement des nanomatériaux fluorescents actuellement.
Synthèse de nanomatériaux fluorescents
Points quantiques (cristaux semi-conducteurs)
Les points quantiques (QD) ont été le sujet de recherche au cours des dernières décennies en raison de leur large spectre d'absorption et de photoluminescence symétrique, de leur rendement quantique élevé, de leur résistance élevée au photoblanchiment, de leurs coefficients d'extinction molaires élevés et de leurs grands décalages effectifs de Stokes [8]. En termes de mécanisme de formation des QD, lorsque les porteurs de charge (électrons et trous) sont limités par des barrières potentielles à certaines régions, les semi-conducteurs présentent des effets de taille quantiques spectaculaires entraînant le décalage du spectre d'absorption et du spectre de fluorescence. Les petites régions sont inférieures à la longueur d'onde de de Broglie des porteurs de charge, ou de manière équivalente, le diamètre du nanocristal est inférieur à deux fois le rayon de Bohr des excitons dans le matériau en vrac [9]. Lorsque les porteurs de charge sont confinés par des barrières de potentiel à trois dimensions spatiales, il se forme des QD, qui sont principalement constitués d'atomes des groupes II-VI (CdSe, ZnS), III-V (GaAs, InP) ou IV-VI (PbS, PbSe).
La synthèse des QDs a été rapportée pour la première fois en 1982 [10, 11]. Des nanocristaux et des microcristaux de semi-conducteurs ont été cultivés dans des matrices de verre. Avec le développement de matériaux fluorescents, les QD ont été préparés par différentes méthodes, telles que la méthode d'adsorption directe, la méthode d'adsorption assistée par linker, les méthodes in situ et la combinaison des méthodes de préparation précédentes. La combinaison des méthodes précédentes comprend la combinaison de semi-conducteurs préparés avec des précurseurs de QD et la combinaison de QD préalablement préparés avec des précurseurs de semi-conducteurs, dans laquelle les semi-conducteurs ou les QD sont préparés séparément [12].
Après une série de recherches sur la synthèse des QD, de nombreux chercheurs ont rapporté l'étude des propriétés fluorescentes des QD. Bawendi et al. synthétisé les QD avec des distributions de taille étroites en introduisant des précurseurs semi-conducteurs tels que le sulfure de cadmium (CdS), le séléniure de cadmium (CdSe) ou le tellurure de cadmium (CdTe) pour étudier les propriétés optiques dépendantes de la taille des QD [13]. Depuis lors, le CdSe est devenu la composition chimique la plus courante des QD, et une variété de modifications de surface [14,15,16] ou de coque inorganique protectrice [13, 17] ont été utilisées pour rendre la stabilité colloïdale.
Points de carbone
Les points de carbone (CD) sont des nanomatériaux émergents de la famille des nanocarbones avec des tailles inférieures à 10 nm, qui ont d'abord été obtenus lors de la purification de nanotubes de carbone à paroi simple (SWCNT) par électrophorèse en 2004 [18]. Il est à noter que les CD remplacent progressivement les points quantiques semi-conducteurs en raison d'une solubilité élevée dans l'eau, d'une faible cytotoxicité, d'une photostabilité élevée, d'une émission multicolore dépendante de l'excitation, d'une flexibilité préférable dans la modification de surface, d'une excellente perméabilité cellulaire et d'une meilleure biocompatibilité [19, 20]. Généralement, les CD comprennent principalement des points quantiques de carbone (CQD) et des points quantiques de graphène (GQD). Une masse de méthodes synthétiques pour les CD avec des tailles ajustables peut être globalement divisée en deux groupes principaux :les méthodes chimiques et les méthodes physiques [21].
Méthodes de synthèse chimique
Les méthodes de synthèse chimique sont les plus couramment utilisées dans la préparation des points de carbone car les CD résultants possèdent d'excellentes propriétés, telles qu'une solubilité dans l'eau supérieure, une inertie chimique, une faible toxicité, une facilité de fonctionnalisation et une résistance au photoblanchiment. En général, les méthodes de synthèse chimique comprennent la synthèse électrochimique [22, 23], l'oxydation acide [24, 25], la carbonisation hydrothermale [26], le traitement par micro-ondes/ultrasons [27, 28, 29], les méthodes de chimie en solution [30], synthèse supportée [31], etc.
Parmi les nombreuses méthodes de synthèse, la synthèse électrochimique a été rapportée à plusieurs reprises au cours des dernières décennies. Le groupe de Zhao a signalé une nouvelle méthode de préparation de CD à faible cytotoxicité par synthèse d'électrooxydation, selon laquelle les CD ont été préparés en oxydant une électrode à colonne graphitique contre une électrode au calomel saturé avec une contre-électrode en fil de Pt dans NaH2 Bon de commande4 solution aqueuse [22]. Le surnageant a ensuite été ultrafiltré à travers des dispositifs de filtration centrifuge pour obtenir les CD avec une fluorescence bleue et jaune, respectivement. Une autre approche électrochimique directe a été récemment rapportée par Qu et al., pour les GQD avec une taille uniforme de 3 à 5 nm par oxydation électrochimique d'une électrode de graphène dans une solution tampon phosphate [23]. La couleur photoluminescente (PL) de ces particules était verte.
Mao et al. a achevé la synthèse d'oxydation par combustion des CD en 2007 en mélangeant de la suie de bougie avec un oxydant, suivi d'un reflux, d'une centrifugation et d'une dialyse pour purifier les CD. Les spectres de photoluminescence des CD préparés ont une large gamme de couleurs, les longueurs d'onde des pics d'émission allant de 415 (violet) à 615 nm (orange-rouge). Ensuite, les CD obtenus ont été soumis à une électrophorèse sur gel de polyacrylamide pour séparer les CD avec différentes caractérisations optiques. L'oxydation acide a également été largement utilisée pour la préparation de nanomatériaux stables tels que les points de carbone. Après le traitement acide des nanotubes de carbone/graphite et le reflux, les CD résultants de 3 à 4 nm présentaient un liquide transparent beige qui émettait une fluorescence jaune vif sous lumière ultraviolette et était assez stable dans une solution saline. Les CD fabriqués avec une fluorescence à ondes longues (jaune/orange/rouge) possèdent une meilleure pénétration. La solution CD peut être conservée à température ambiante pendant une longue période et aucun précipité ne se forme provoquant la perte de fluorescence [25].
La synthèse par micro-ondes/ultrasons est progressivement et principalement devenue une technologie de synthèse auxiliaire dans le processus de synthèse [32]. Des CD fluorescents, de 3 à 5 nm de diamètre, ont été synthétisés par le groupe de Xiao via une approche assistée par micro-ondes économique, rapide et verte [33]. La caractéristique la plus marquante de cette approche en une étape était que la formation et la fonctionnalisation des CD ont été achevées simultanément par la pyrolyse par micro-ondes dérivée des liquides ioniques pour la première fois [34]. Le processus de réaction s'est produit dans un four à micro-ondes utilisant des liquides ioniques bon marché comme source de carbone et la solution est passée de l'incolore au brun foncé au fil du temps de réaction [35]. Tang et al. ont utilisé une méthode par ultrasons sur la base de glucose ou de charbon actif comme source de carbone pour synthétiser des CD hydrosolubles monodispersés. Ils ont émis une fluorescence lumineuse et colorée [28]. De même, Vanesa Romero et al. obtenu des points de carbone (CD) co-dopés à l'azote (N) et au soufre (S) hautement fluorescents après oxydation photochimique des glucides dans les légumes. Le codopage de N et S augmente le nombre de sites actifs à la surface des CD, améliorant ainsi ses performances de luminescence [36]. Les points quantiques de carbone dopés à l'azote (NCQD), une sonde fluorescente, ont été appliqués avec succès à la détermination de la doxycycline [37]. Pathak et al. ont également préparé des points de carbone co-dopés avec de l'azote et du soufre (NSCD), qui ont été synthétisés à partir de tampon thiourée et tris-acétate-éthylènediamine par la méthode hydrothermale micro-ondes. Les NSCD ont été utilisés pour imager diverses bactéries pathogènes et cellules épithéliales buccales humaines en raison de la fluorométrie multicolore [38].
Considérant que la plupart des méthodes synthétiques mentionnées ci-dessus nécessitaient un acide fort, plusieurs étapes expérimentales compliquées et d'autres modifications avec d'autres composés pour améliorer la solubilité dans l'eau des CD et améliorer leur propriété de photoluminescence, certaines équipes de recherche ont exploité la carbonisation hydrothermale de la photoluminescence des glucides tels que chitosane, glucose, acide citrique, etc. pour éviter les processus complexes et longs de purification et de fonctionnalisation [39]. Yang et al. ont décrit une méthode synthétique en une étape pour les CD fluorescents hautement fonctionnalisés en amino avec un rendement quantique (QY) de 7,8 % par carbonisation hydrothermale du chitosane à une température douce. Cette méthode ne nécessitait ni solvant acide fort ni réactif de passivation de surface. En outre, les groupes fonctionnels à la surface des CD ont amélioré leur solubilité dans l'eau et réduit leur biotoxicité potentielle [26]. Les points de carbone multi-dopés (MCD), avec une émission lumineuse et réglable en couleur, ont été synthétisés par la méthode à un pot sans aucune passivation de surface supplémentaire. Les MCD synthétisés ont été dopés avec des éléments biogéniques abondants (O, N, P) et présentent donc une forte émission fluorescente et une caractéristique dépendante de la longueur d'onde d'excitation, une bonne solubilité aqueuse, une stabilité optique élevée ainsi qu'une stabilité ionique. Les MCD peuvent non seulement détecter de manière sélective et sensible Fe 3+ sous lumière bleue de détection à 15,9 nm, mais aussi mesurer le Fe 3+ intracellulaire par imagerie par fluorescence multicolore [40].
Pour les méthodes de chimie en solution, la condensation oxydative des groupes aryle a été appliquée avec succès à la préparation de GQD au cours des dernières décennies. Des GQD colloïdaux stables avec les tailles et les structures souhaitées ont été produits par le groupe de Li avec une stratégie de solubilisation. Cette méthode a permis d'obtenir une accordabilité de taille et une distribution de taille étroite des CD sans aucun processus de séparation de taille peu pratique [30]. En ce qui concerne la procédure de synthèse soutenue, plusieurs équipes de recherche en ont profité pour compléter la synthèse de nanomatériaux monodispersés tels que les CD nanométriques. Le groupe de Zhu a adopté des sphères de silice mésoporeuse (MS) comme nanoréacteurs et de l'acide citrique comme précurseur de carbone et des CD hydrophiles d'une taille de 1,5 à 2,5 nm ont été préparés par une méthode d'imprégnation. Les CD avec une efficacité de photoluminescence élevée de 23 % étaient capables d'émettre une forte luminescence bleue et de présenter d'excellentes propriétés de luminescence de conversion [31]. Des points de carbone émissifs jaune vif (Y-CD) ont été préparés par Yan et al. par la méthode solvothermique, en utilisant l'acide citrique anhydre comme source de carbone et la 2,3-phénazinediamine comme source d'azote. Les Y-CD avec des groupes carboxyle abondants ont affiché un rendement quantique de fluorescence respectable (24 %), un décalage de Stokes à 188 nm, une sensibilité élevée et une excellente stabilité [41]. Les méthodes de synthèse et les propriétés des CD sont présentées dans le tableau 1.
Méthodes de synthèse physique
En général, les méthodes de synthèse physique comprennent principalement la décharge à l'arc, l'ablation/passivation au laser et le traitement au plasma. Xu et ses collègues ont oxydé la suie de décharge d'arc avec du HNO3 puis séparé la suspension par électrophorèse sur gel en SWCNT. Ils ont finalement isolé la bande à déplacement rapide de nanoparticules de points de carbone hautement fluorescentes [18]. Des CD utilisant des matériaux nanocarbonés comme précurseur et un solvant respectueux de l'environnement comme milieu liquide ont été préparés par Li et al. via une approche d'ablation laser douce [44]. De plus, Gokus et ses collègues ont démontré que l'utilisation d'un plasma d'oxygène pouvait induire une forte fluorescence dans le graphène monocouche [45].
Nanoparticules de carbone
Les nanoparticules de carbone fluorescentes, avec leur cytotoxicité réduite, leur résistance au photoblanchiment et leur biocompatibilité accrue, attirent de plus en plus l'attention pour la bio-imagerie et d'autres applications biomédicales. Par rapport aux tailles typiques des points de carbone entre 1 et 6 nm, les tailles des nanoparticules de carbone sont supérieures à 20 nm, ce qui évite les problèmes de séparation, de purification et de collecte [46]. Les méthodes de synthèse des nanoparticules de carbone sont similaires aux points de carbone, notamment la carbonisation hydrothermale, le traitement par micro-ondes, la méthode d'ablation chimique et l'ablation laser. Ces méthodes ont leurs propres avantages mais ne permettent pas de contrôler efficacement la taille des nanoparticules. La carbonisation électrochimique est une méthode en une seule étape qui permet de contrôler la taille et les propriétés de luminescence des nanoparticules de carbone. Malheureusement, il n'y a que très peu de substrats disponibles pour cette méthode. À l'heure actuelle, de nouvelles méthodes intrigantes ont été signalées, telles que la méthode de combustion au pentoxyde de phosphore [47].
Ces dernières années, des nanoparticules de carbone adaptées aux applications biomédicales ont été synthétisées avec des méthodes modifiées. Santu et al. ont résolu la synthèse de nanoparticules de carbone fluorescentes rouges de haute qualité par carbonisation contrôlée du résorcinol [48]. Cette approche implique un couplage oxydant du phénol associé à une déshydratation pour former des nanoparticules de carbone fluorescentes rouges. Anara et al. synthétisé des nanoparticules de carbone fluorescentes avec un rendement quantique de 6,08 % en utilisant une méthode hydrothermale modifiée. Par rapport aux méthodes conventionnelles qui nécessitent un traitement thermique long jusqu'à plusieurs heures, cette méthode a raccourci le temps de réaction à moins de 30 min, réalisant la synthèse rapide de nanoparticules de carbone fluorescentes [46].
Nanotubes de carbone
Les nanotubes de carbone unidimensionnels (1D) ont suscité une énorme attention dans le domaine biomédical en raison de leurs excellentes propriétés électroniques et optiques. Les nanotubes de carbone peuvent être divisés en nanotubes de carbone monoparoi (SWCNT) et nanotubes de carbone multiparois (MWCNT) selon le nombre de couches cylindriques de graphène. Alors que les SWCNT sont composés d'une seule couche de feuille de graphène enroulée dans un cylindre, les MWCNT comprennent plusieurs couches concentriques de feuille de graphène. Le diamètre extérieur des nanotubes de carbone est inférieur à 100 nm, mais leurs longueurs peuvent atteindre plusieurs millimètres, ce qui conduit à un rapport d'aspect très élevé et à une grande surface [49]. De plus, l'arrangement unique des atomes de carbone dans les nanotubes de carbone forme une riche conjugaison d'électrons à l'extérieur du nanotube [50]. De plus, les nanotubes de carbone sont dotés d'une forte absorption et fluorescence dans la région NIR [51]. Toutes ces caractéristiques contribuent à une interaction efficace avec les biomolécules, ce qui fait des nanotubes de carbone un candidat idéal pour les applications biomédicales.
Les méthodes synthétiques ont une grande influence sur le diamètre, la longueur, la structure, la chiralité et la qualité des nanotubes de carbone, et en attendant, il convient d'examiner si cette méthode se prête à une production à grande échelle. Les méthodes couramment utilisées comprennent la décharge à l'arc [52], l'ablation au laser [53] et le dépôt chimique en phase vapeur [54]. De plus, les nanotubes de carbone doivent être fonctionnalisés pour améliorer leur solubilité et éviter leur agrégation dans les solvants et les milieux biologiques. La fonctionnalisation covalente introduirait des défauts dans la structure des nanotubes de carbone, entraînant une diminution spectaculaire voire une perte complète de leur fluorescence NIR. La fonctionnalisation non covalente avec des molécules amphiphiles telles que des polymères préserverait la structure et les propriétés fluorescentes des nanotubes de carbone, mais abaisserait le QY des nanotubes de carbone. Afin de surmonter ces obstacles, de nouvelles méthodes de synthèse et de fonctionnalisation des nanotubes de carbone ont été récemment rapportées. Lee et al. ont rapporté que l'ajout de dithiothréitol, qui est un agent réducteur, peut améliorer le QY fluorescent des SWCNT pour la première fois, résultant en des fluorophores ayant une luminosité équivalente à celle des QDs [55]. Hou et al. ont étudié l'ajout de dithiothréitol aux SWCNT fonctionnalisés avec une variété de tensioactifs. Pour les SWCNT enveloppés d'ADN et de SDS, leur QY fluorescent a augmenté de manière significative, tandis qu'une extinction de la fluorescence à différents degrés a été observée pour d'autres surfactants [56]. En conséquence, l'ajout de dithiothréitol à l'ADN ou aux SWCNT enveloppés de SDS sont des solutions réalisables pour réaliser l'application de nanotubes de carbone en biomédecine.
Nanomatériaux à base de graphène
En tant que nanomatériaux carbonés bidimensionnels, le graphène et ses dérivés ont été largement explorés pour une gamme d'applications biomédicales telles que la bioimagerie et l'administration de médicaments. Les nanomatériaux de graphène comprennent la nanofeuille de graphène, l'oxyde de graphène (GO) et la nanofeuille d'oxyde de graphène réduit (rGO). Ils ont des surfaces élevées et des propriétés de surface uniques qui permettent des interactions non covalentes avec des molécules de colorant, des biomolécules et des médicaments insolubles dans l'eau. De nombreux chercheurs ont signalé différentes méthodes de préparation du graphène depuis qu'il a été préparé avec succès pour la première fois en 2004. Les méthodes de synthèse des nanomatériaux de graphène peuvent être classées en deux catégories, descendante et ascendante.
Les méthodes descendantes impliquent l'isolement des couches de graphite empilées pour former des feuilles de graphène, y compris l'exfoliation mécanique [57], l'exfoliation à base de solvant [58] et l'exfoliation électrochimique [59]. Gu et al. ont systématiquement étudié l'exfoliation à base de solvant assistée par ultrasons et ont constaté que les ondes ultrasonores ont un bon effet d'exfoliation. Ils peuvent également affecter la distribution de la taille et de l'épaisseur des feuilles de graphène, ce qui permet une synthèse contrôlable. Les approches ascendantes impliquent la réorganisation des atomes de carbone en utilisant des sources de carbone alternatives. La croissance épitaxiale [60] et le dépôt chimique en phase vapeur (CVD) [61] sont les méthodes de synthèse ascendantes les plus couramment utilisées. Feuilles GO composées de plusieurs sp 2 les domaines isolés par des groupes contenant de l'oxygène peuvent être synthétisés en utilisant la méthode de Hummer. Variations de tailles de ces sp 2 les domaines font que le PL des feuilles GO varie largement de 500 à 800 nm [62]. rGO est dérivé de GO par réduction chimique à l'aide d'agents réducteurs tels que l'hydroquinone et l'hydrazine. Par rapport à GO, la fluorescence de rGO a montré une émission décalée vers le bleu dans la région UV avec une extinction de fluorescence, qui est attribuée aux voies de percolation entre le sp 2 cristallin nouvellement formé grappes [63]. Akbari et al. élucidé que le rapport de sp 3 /sp 2 domaines dans les feuilles GO détermine leurs spectres de fluorescence. Par conséquent, GO est un nanomatériau fluorescent prometteur sur une large gamme de longueurs d'onde sous différents degrés de réduction, qui peut être utilisé dans les applications biomédicales.
Nanomatériaux métalliques
Les atomes de métaux nobles présentent en même temps moins de cytotoxicité que les QDs. Les nanoparticules d'or, d'argent et de cuivre font l'objet d'une attention croissante et sont appliquées à un grand nombre de domaines. Dans les domaines biomédicaux, les effets mécaniques quantiques des nanoparticules d'or, tels que l'émission de photoluminescence ou la résonance plasmonique, font des nanoparticules d'or (AuNP) un candidat idéal pour un autre nanocapteur in vivo à faible cytotoxicité [64, 65].
Les AuNP ont suscité un grand intérêt scientifique en raison de leur facilité de synthèse et de leurs propriétés uniques, et diverses méthodes de synthèse ont été rapportées. En tant que l'une des méthodes les plus importantes, les méthodes chimiques sont généralement effectuées en traitant une solution aqueuse de chloroaurate avec des agents réducteurs en présence d'un agent stabilisant. L'acide citrique est surtout largement utilisé, qui peut agir à la fois comme stabilisant et agent réducteur [66]. Cependant, les AuNPs stabilisées avec de l'acide citrique peuvent subir une accumulation irréversible au cours du développement de la fonctionnalisation avec des ligands thiolates. Ce problème peut être surmonté en faisant en sorte que la réaction ait lieu en présence de polymères solubles dans l'eau, de tensioactifs ou d'agents de coiffage qui aident à fournir une stabilité plus élevée et à empêcher l'agrégation des nanoparticules. La taille et la forme des AuNPs peuvent être contrôlées en modifiant la proportion or-citrate, les agents modificateurs de surface ou les conditions de réaction. Avec la méthode d'émulsification par ultrasons en un seul pot, Zhang et ses collègues ont co-chargé du Bis(4-(N-(2-naphtyl)phénylamino)phényl)-fumaronitrile et des AuNPs dans des micelles pour obtenir la nanosonde [67]. Surtout, la nanosonde obtenue, avec un grand potentiel d'application dans l'imagerie ciblée sur les tumeurs et le diagnostic in vivo, a traité une excellente capacité d'imagerie par fluorescence, malgré l'existence de nanoparticules d'or. Bien que les AuNPs ne soient pas toxiques dans certaines conditions expérimentales, la toxicité et les effets secondaires doivent être soigneusement examinés [68].
Les nanoclusters d'Ag fluorescent ont reçu beaucoup d'attention en raison de leurs propriétés physiques et chimiques uniques. Le processus de synthèse de ces nanoclusters est classé par l'échafaudage stabilisant en oligonucléotides d'ADN, peptides, protéines, dendrimères et polymères. En outre, une littérature abondante a démontré une certaine synthèse verte, telle que l'application d'extraits aqueux de tige de D. trifoliata et S. alba pour optimiser les conditions de préparation [69].
Les nanoclusters de Cu (Cu NCs) sont relativement largement utilisés comme matériaux de métaux nobles, mais leur synthèse est encore rare en raison de leur vulnérabilité à l'oxydation. Récemment, Kawasaki et al. préparé avec succès des NC Cu stables par une méthode polyol assistée par micro-ondes [70]. L'ADN pourrait être utilisé comme matrice pour la synthèse de NC Cu fluorescentes. Mohir et al. ont proposé une méthode basée sur l'ADN double brin en solution pour obtenir des NC Cu avec une sélectivité élevée [71]. En utilisant les propriétés fluorescentes des NC Cu, il a été exploité avec succès comme indicateur de signal d'activation fluorescent efficace pour la détermination sélective de RDX [72].
Nanoparticules de silice
Compte tenu des propriétés de transparence, de stabilité mécanique, de robustesse et de stabilisation des fluorophores intégrés, les nanoparticules de silice sont largement appliquées dans les domaines biologiques. Par exemple, les NP à noyau/enveloppe de silice appliquées à la détection intracellulaire de Zn 2+ et H2 Bon de commande4 − dans des cellules vivantes ont été synthétisés sous forme de nanocapteurs fluorescents « off/on ». Ces dernières années, des nanoparticules de silice dopées avec des colorants organiques ont été synthétisées et largement utilisées dans de nombreuses applications telles que la biodétection [73]. Les méthodes de synthèse les plus utilisées pour les nanoparticules de silice sont la méthode Stöber et la méthode de microémulsion inverse. La méthode Stöber, décrite pour la première fois dans les années 1960 [74], implique l'hydrolyse de silicates d'alkyle et la condensation subséquente d'acide silicique dans des solutions alcooliques catalysées par l'ajout d'ammoniac. La deuxième méthode de formation de NP de silice, la méthode de microémulsion inverse, implique la réaction de silicates d'alkyle, typiquement TEOS, à l'intérieur des gouttelettes d'eau d'une microémulsion eau-dans-huile [75]. Il et al. préparé trois types de nanoparticules de silice de dopage de colorant avec la méthode Stöber et la méthode de microémulsion inverse en incorporant un colorant dans le noyau de la particule. Certaines molécules fonctionnellement sensibles au Zn 2+ sont déposés sur les surfaces des particules [76]. Les nanoparticules de silice fluorescente ont été utilisées pour des images fluorescentes intracellulaires Zn 2+ (H2 Bon de commande4 − ) dans les cellules HeLa. Lorsque Zn 2+ a été ajouté au Zn 2+ proportionnel nanocapteur, les nanoparticules ont montré la capacité de détecter de manière ratiométrique la concentration de H2 Bon de commande4 − .
En général, les nanocomposites de silice avec une bonne monodispersité et biocompatibilité peuvent être facilement modifiés davantage avec des groupes fonctionnels [77,78,79]. Lee et al. nanoparticules magnétiques dopées et colorants fluorescents en nanoparticules de silice. Ces nanocomposites de silice peuvent être utilisés non seulement comme sondes d'imagerie multimodales pour l'imagerie par résonance magnétique (RM) et par fluorescence, mais également comme vecteurs d'administration de médicaments anticancéreux [80]. En un mot, les particules de silice pourraient être un sujet de recherche dans de tels cas avec des utilisations très étendues.
Phosphores
Les phosphores sont largement utilisés en biomédecine en raison de leurs avantages uniques dans la réduction de l'autofluorescence et des interférences de diffusion de la lumière des tissus. En général, les luminophores sont composés de matériaux hôtes et d'ions dopés [81]. Parmi les matériaux hôtes des luminophores, l'oxyde d'yttrium (Y2 O3 ) est plus que prometteur en raison de sa faible photodurabilité et de son énergie de phonons. Les lanthanides sont largement dopés en phosphores compte tenu de leurs niveaux d'électrons abondants et de leurs canaux de transfert d'énergie. De nombreuses méthodes ont été décrites pour la préparation de luminophores, notamment les procédés hydrothermaux [82], pyrolyse par pulvérisation à la flamme [83], sol-gel [84] et de co-précipitation [85].
La synthèse hydrothermale est en train de devenir un processus idéal, qui s'est avéré efficace et économique pour la synthèse de luminophores. Yu et al. synthétisé Y2 O3 :Eu 3+ phosphores par voie hydrothermale en présence de citrate de sodium [82]. Le concentré de citrate de sodium, la quantité ajoutée de NaOH et d'Eu dans le processus hydrothermal ont décidé des propriétés des luminophores obtenus. La pyrolyse par pulvérisation à la flamme est une méthode prometteuse pour la synthèse rapide et consécutive de phosphores à base d'oxyde. Par rapport aux méthodes conventionnelles, cette méthode fournit des luminophores avec une cristallinité élevée et une distribution homogène des dopants. Khan et al. produit avec succès Tb 3+ –dopé Y2 O3 phosphores d'environ 100 nm de diamètre avec une distribution de taille étroite utilisant la pyrolyse par pulvérisation à la flamme [83]. Dans leur méthode, le sel alcalin a été mélangé avec d'autres précurseurs de nitrate de métal, qui contrôlaient efficacement la distribution granulométrique dans une plage étroite. The sol–gel synthesis route offers several advantages, such as high homogeneity and purity, reduced synthesis time, uniform particle morphology, and narrow particle size distribution [86]. Leonardo et al. obtained Sm 3+ doped SiO2 -Gd2 O3 phosphors by a sol–gel process [84]. Co-precipitation is a common and simple method for synthesizing crystalline phosphors, which ensures high homogeneity and controlled morphology characteristics. Perhaita et al. reported that the phase composition of the phosphors strongly depends on the pH during the precipitation [85].
Organic Frameworks
Covalent-organic frameworks (COFs) are new porous crystalline materials possessing outstanding stability, adsorption and low toxicity. The design of fluorescent small organic molecules with a combination of fluorescence determination methods can be used to construct more efficient nanoprobes [87]. For selective 2,4,6-trinitrophenol (TNP) determination, a novel Naphthalimide-Benzothiazole conjugate was prepared as colorimetric and fluorescent nanoprobe. The fluorescence emission peaks of receptor were selectively quenched by TNP with a limit of detection as low as 1.613 × 10 –10 M.
Metal organic frameworks (MOFs) are a kind of new generation multifunctional inorganic–organic materials with various holes and functionalized 3D crystalline structures formed by metal ions and linkers. MOFs show potential applications in separation, catalysis and other aspects due to unique attributes such as excellent chemical tenability, specific surface area and confinement of the pores. Some of the MOFs are luminescent and the quantum yield as well as light intensity will be influenced by temperature and excitation wavelength [88]. With the addition of doxycycline, Yu et al. synthesized a new functional metal–organic framework of pyromellitic acid and europium, which exhibited remarkable fluorescence enhancement at 526 nm and 617 nm. Results showed that both fluorescence intensities were positively correlated with the doxycycline concentration. The unique fluorescence response of the system could discriminate doxycycline from other tetracycline antibiotics with high selectivity.
Biomedical Applications of Fluorescent Nanomaterials
Bioimaging
Quantum Dots for Bioimaging
Fluorescent nanomaterials have been widely used in bioimaging. Compared with conventional organic fluorescent molecules, fluorescent nanomaterials are equipped with many superior properties such as high photostability, tunable emission spectra and high quantum yields [89].
As early as 1998, QDs were first successfully applied in biological imaging [90]. Since then, applications of QDs in this field have been springing up gradually. Chen’s group applied it in bioimaging and nuclear targeting with great stability and biocompatibility in living cells [91]. In spite of the extremely high sensitivity and spatial resolution of QDs, poor performances on hydrophilicity and biocompatibility hindered their applications in bioimaging in vivo. To tackle this problem, it has been found that the water-solubility of QDs can be greatly improved by attaching thiol or other hydrophilic groups to the surface of quantum dots [92]. In the same way, with the intention of improving the effectiveness and specificity of in vivo targeted imaging, targeting molecules are attached to the surface of QDs. Furthermore, the wavelength region of the emission light can be controlled by altering the size of QDs.
The combination of QDs and inorganic metal ions can optimize the application of QDs in bioimaging because the QDs’ defect-site PL peaks will be utterly removed by controlling the proportion of doped inorganic metal ions. Kuwabata, S et al. modulated the degree of Ga 3+ doping in Ag–In–Se QDs. Thus, the QDs’ defect-site PL peaks were completely removed and a sharp band-edge emission peak come into appearance [93]. They found a blue shift of the band-edge PL peak ranging from 890 to 630 nm which could be credited to the fact that the energy gap of QDs was enlarged by Ga 3+ doping. After injecting a mouse with QDs, the potential of AIGSe@GaSx core–shell QDs for bioimaging turned out satisfying. The imaging effect of this kind of QDs in mice is demonstrated in Fig. 2. However, sensing of mid-IR wavelengths is challenging due to increased dark currents and noise. HgTe QDs synthesized by colloidal method is a promising candidate for IR bioimaging by virtue of lower dark currents, higher-temperature operation, and higher detectivity [94].
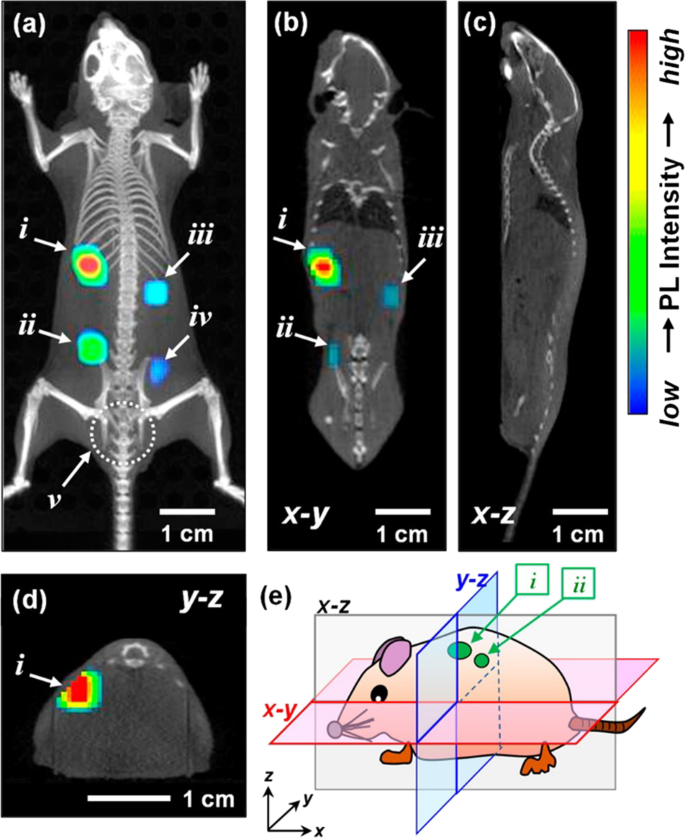
Three-dimensional PL image superimposed on an X-ray CT image of the mouse subcutaneously injected with DSPC-AIGSe@GaSx liposome dispersions (each 50 mm 3 ) in the back [78]
Carbon Dots for Bioimaging
The poor photostability of current fluorescent nanomaterials hinders their long-term bioimaging to a large extent. To overcome this limitation, CDs have been studied for bioimaging and some positive results have been obtained due to the great performance on PL efficiency. Enormous efforts have been put to improve their water solubility and lower their toxicity in organisms. At present, most CDs are facing a barrier in bioimaging, that is, their short-wavelength excitation disables deep penetration in tissue. Aside from this, being exposed under the short-wavelength for a long time could do irreversible damages to living cells and tissues. As shown in Fig. 3, with the purpose of overcoming this deficiency, Gao et al. designed fluorescent CDs with red emission which were successfully used for bioimaging of noble metal ions (Pt 2+ , Au 3+ , Pd 2+ ) in cells and zebrafish [95]. Sun and co-workers first studied the near infrared (NIR) imaging of CDs in vivo using mice as a model. Recently, it was reported that molecules or polymers containing plentiful sulfoxide or carbonyl groups can enhance NIR fluorescence through the surface modification. As shown in Fig. 4, under NIR excitation, sulfoxide or carbonyl groups are bound to the outer layers and the edges of the CDs. Thus, electron transitions are promoted, influencing the optical bandgap [96].
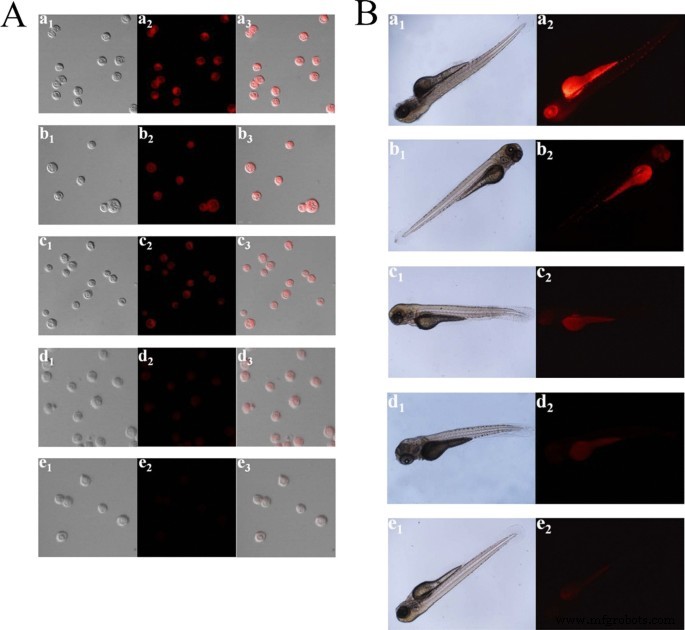
A Confocal imaging of Pt 2+ in PC12 cells. (a1–e1) Bright field images. (a2–e2) Black field images of the CDs in PC12 cells with the different concentrations of Pt 2+ (0, 25, 50, 150, and 300 μM). (a3–e3) Overlay images. B Fluorescence imaging of Pt 2+ in ZF. (a1–e1) Bright field images. (a2–e2) Fluorescence images of the CDs in ZF with the various concentrations of Pt 2+ (0, 30, 60, 100, and 150 μM) [80]
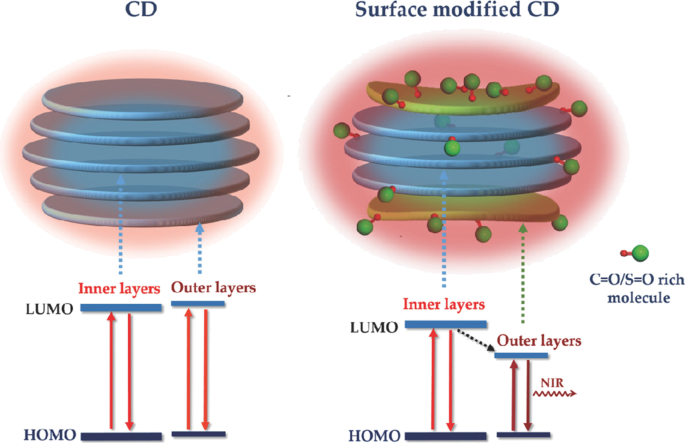
Schematic of structure and energy level alignments of nontreated CDs (left column) and CDs modified with S = O/C = O‐rich molecules (right column). The red (oxygen atom) and green double‐bonded balls represent the C = O/S = O‐rich molecule [81]
Carbon Nanoparticles for Bioimaging
In the field of bioimaging, fluorescent carbon nanoparticles show unique chemical and optical properties over traditional fluorescence probes. Different size, shape and elemental composition make carbon nanoparticles with different features. The biomedical fields are always seeking the most promising fluorescent carbon nanoparticles. Gaurav et al. obtained both larger and smaller size carbon nanoparticles with laser ablation method [97]. Both green and blue fluorescence were observed in the cells incubated with the carbon nanoparticles, suggesting their different sizes. Cell viability results indicated that the prepared carbon nanoparticles were nontoxic and safe for bioimaging applications. Shazid et al. employed carbonization method to obtain fluorescenct carbon nanoparticles derived from biocompatible hyaluronic acid. Both the in vitro and in vivo bioimaging studies showed that the prepared carbon nanoparticles would be reliable and stable for opticle imaging. Moreover, based on the experimenal data, their cytotoxicity was proved to be tolerable for biomedical applications.
Carbon Nanotubes for Bioimaging
Fluorescence of carbon nanotubes in the NIR is attracting high attention for their good light penetration depth in biological tissues. However, their low quantum yield requires for considerable excitation doses, leading to a fair degree of blue-shift and failure of penetrating live tissue. Mandal et al. reported that bright and biocompatible p-nitroaryl functionalized SWCNTs, encapsulated in phospholipid-polyethylene glycol, are suitable for bioimaging applications. The prepared SWCNTs enabled high signal-to-noise ratio imaging in live brain tissues using ultra-low excitation intensities. Their 1160 nm emissions in the NIR guarantee that they will provide optimal fluorescence imaging results [98]. Ceppi et al. applied SWCNT-based fluorescence imaging to debulking surgery in an ovarian cancer mouse model. SWCNTs are coupled to an M13 bacteriophage carrying modified peptide binding to the SPARC protein, which is overexpressed in ovarian cancer, leading to real-time imaging to guide intraoperative tumor debulking. This imaging system enables detection in the NIR window with a pixel-limited resolution of 200 μm, demonstrating real potential in fluorescence imaging guided surguries for patients [99].
Furthermore, fluorescent moieties can be conjugated by a carbon nanotube backbone, which integrate strong fluorescent ability with robust mechanism strength, exhibiting ideal bioimgaing results. Katharina et al. functionalized SWCNTs with an amphiphilic C18 -alkylated polymer conjugated with bright perylene bisimide fluorophores. The polymers wrapping around the SWCNT backbones not only increase their water dispersibility but also promote their biocompatibility by providing a shield. In vitro studies on HeLa cells demonstrated that the biocompatibility of SWCNTs is dramatically improved. In microscopy studies, direct imaging of the SWCNTs' cellular uptake via perylene bisimide and SWCNT emission proved their potential for bioimging [100]. Park et al. combined carbon nanotubes with mussel adhesive proteins which can be specifically targeted at tumors in tissue. They then made carbon nanotubes conjugated with a ZW800 NIR fluorophore to obtain NIR fluorescence imaging [101]. The prepared carbon nanotube probes react with a specific tumor in one hour and can be easily eliminated via urine, demonstrating great value as tumor imaging and detecting agent.
Graphene-Based Nanomaterials for Bioimaging
Large surface area and feasible further functionalization make graphene-based nanomaterials a promising candidate for biomedical applications. However, as a result of their chemical sturctures, graphene nanosheets lack photoluminescence and rGO only display weak fluorescence, which makes it difficult to be utilized in bioimgaing applications. Many researchers attempted to resolve this problem by conjugation of fluorescent dyes and probes onto the large surface of graphene and its derivatives. Sun et al. reported an assembly strategy to prepare fluorescence probe RACD functionalized a single layer GO via π-π interaction and hydrogen bonding. The resluting nanomaterials exhibited that the fluorescent probes reduce the aggregation degree and acquire very well monodispersion, hydrophilicity and photostability, which is attributed to the strong synergy between RACD and GO [102]. Even so, fluorescence quenching remains a critical issue for these materials. In addition, the biocompatibility and toxicity of polymers applied to connect graphene-based materials and fluorescent moieties have not been adequately investigated. These facts appeal for alternative solutions to utilize graphene and its derivatives in bioimaging applications. Georgia et al. developed intrinsically photoluminescent graphene derivatives that show desirable biocompatibility and tunable fluorescence properties [103]. They can be organophilic or hydrophilic with different amine functionalization dodecylamine and hexamethylenediamine, respectively. The intrinsic fluorescent graphene-based nanomaterials possess great potential in a variety fileds of bioimgaing.
Metal Nanomaterials for Bioimaging
In recent years, fluorescent metal nanoparticles have shown great potential in bioimaging for improved disease diagnosis and treatment [104]. Gold is the most commonly used metal for bioimaging. The surface of AuNPs can be easily modified with various biomolecules such as peptides, proteins, antibodies, enzymes, and nucleic acids. These biomolecules can interact with specific cells or organelles in vivo, which makes it possible for AuNPs to be used for targeted optical imaging. Gao et al. reported a real-time in situ imaging of nucleus by AuNPs fabricated with bifunctional peptides constructed with both Au-binding affinity and nucleus-targeting ability. The bifunctional peptides showed strong binding affinity toward AuNPs and ensured good surface coverage of the nanoparticles, which made it stable and efficient for precise bioimaging of the nucleus in cells [105]. The Au-Se bond is considered as a better candidate than the Au–S bond to link the peptides and AuNPs due to the stronger ability against interference of intracellular thiol. Pan et al. prepared the Au-Se-peptide nanoprobes through a direct freezing process. The obtained nanoprobe was successfully applied to identify autophagy and apoptosis in chemotherapeutic drug treated cancer cells [106].
As a novel fluorescent imaging technology, DNA-templated silver nanoclusters (DNA-Ag NCs) have aroused the attention of many scientists due to their unique properties, especially the tunable fluorescence emission range relying on DNA sequences. However, the highly negatively charged DNA backbones have always been a great obstacle for the expansive applications in bioimaging because of poor stability as well as poor cell permeability in physiological environment. It is also noteworthy that the PL property and fluorescent efficiency of DNA-Ag NCs are far from satisfying. As a result, figuring out how to neutralize the negative charge on the surface of DNA strands is of great urgency for researchers. Recently, Lyu and co-workers successfully modified fluorescent DNA-Ag NCs with cationic polyelectrolytes via electrostatic force between the positively charged polyelectrolytes and the negatively charged phosphate groups of the DNA strands, leading to a threefold fluorescence intensity enhancement [7] (Fig. 5). Li et al. reported a facile strategy to make gold nanoclusters with positive charge and silver nanoclusters with negative charge form aggregates by electrostatic interactions. An incredible 40-fold fluorescence intensity enhancement was obtained. Results demonstrated that the physiological stability improved a lot and the cell permeability was also enhanced, which promises its practical applications in the future.
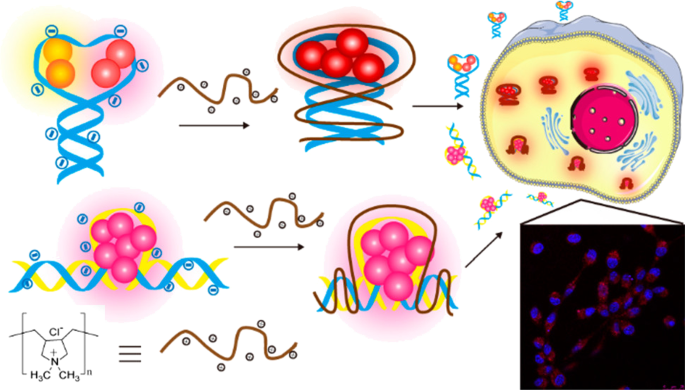
Formation of FL DNA–Ag NC–Cationic Polyelectrolyte Complexes for Cell Imaging [7]
Silica Nanoparticles for Bioimaging
Dye-doped fluorescent silica nanoparticles emerge with great potential for bioimaging as a novel and ideal platform for the monitoring of living cells and the whole body. The outer silica shell matrix protects fluorophores from outside chemical reaction factors as well as provides a hydrophilic shell for the inside insoluble nanoparticles, which renders the enhanced photo-stability and biocompatibility to the organic fluorescent dyes. Benefiting from the robust structure of silica matrices, dye-doped fluorescent silica nanoparticles have been presented with several superior properties including good biocompatibility, hydrophilic features, and high fluorescence intensity [107].
Jiao et al. also constructed a local hydrophobic cage in dye-doped fluorescent silica nanoparticles to improve their optical properties, which solves the problems of aggregation-caused quenching (ACQ) and poor photostability in aqueous media by organic fluorescence dyes benefiting from the robust structure of silica nanoparticles [108]. In addition, compared with free dyes, the fluorescent intensity both in water solution and living cells demonstrated a 12.3-fold enhancement due to the limitation of molecular motion, indicating a significant development for silica nanoparticles in biomedical applications. QDs have been developed for bioimaging both in vivo and vitro owing to their excellent optical qualities. However, a critical obstacle faced in QDs’ application in vivo is their poor biocompatibility. Inspired by the organic dye-conjugated silica-NPs, QDs-embedded silica-NPs have also been invented with the advantage that the excellent optical qualities of QDs can be retained, while the silica-NPs coat improves their biocompatibility to a large extent simultaneously. Darwish et al. reported that many QDs could be assembled around a central silica nanoparticle to form supra-NP assemblies. It was expected to be used for enhanced bioimaging because of their higher sensitivity and superior signal-to-background ratios [109]. There is reason to believe that silica-NPs conjugated with fluorescent nanomaterials with ideal optical properties will still be the dominant research interest in the future.
Phosphors for Bioimaging
For bioimaging, the sizes of phosphors need to be controlled so that they are small enough to be integrated with living cells. Furthermore, the aggregation of particles should be avoided for biocompatibility. Hence, the control of both particle sizes and dispersity in an aqueous solution is essential for the bioimaging application of the phosphors. Atabaev et al. prepared Eu, Gd-codoped Y2 O3 phosphors which had a spherical morphology within the range 61–69 nm. Enhanced PL emission and low toxicity made these phosphors suitable for bioimgaing applications [110].
Upconversion nanomaterials are able to convert lower-energy near-infrared photons to higher-energy ones as emission. This anti-Stokes photoluminescence process will lead to low background noise, large tissue penetration depth, and low photo-damage in bioimaging applications [111]. Lanthanide-based phosphors are able to show upconversion emission owing to their photodurability and low phonon energy. Nallusamy et al. reported a NIR–NIR bioimaging system based on Er 3+ :Y2 O3 phosphors by using NIR emission at 1550 nm under 980 nm excitation, which can allow a deeper penetration depth into biological tissues than ultraviolet or visible light excitation [112]. In addition, the surface of Er 3+ :Y2 O3 was electrostatically PEGylated to improve the chemical durability and dispersion stability under physiological conditions. Thakur et al. synthesized Ho 3+ /Yb 3+ co-doped GdVO4 phosphors via a modified sol–gel method. The prepared phosphors showed brilliant red upconversion emission under NIR excitation, which may be useful in bioimaging of the biomolecules [113].
Organic Frameworks for Bioimaging
Careful selection of MOF constituents can yield crystals of ultrahigh porosity and high thermal and chemical stability, with some of them being luminescent [114]. Recently, Sava Gallis’s group described a novel multifunctional MOF material platform which showed a wide spectral region from 614 to 1350 nm covering the deep red to NIR region. Both porosity and tunable emission properties made them highly suitable for in vivo bioimaging [115]. What’s more, to overcome the obstacle of MOF’s low selectivity towards malignant tissues, Liu et al. developed a target-induced bioimaging by conjugating DNA aptamers using ZrMOF nanoparticles as quenchers [116]. Based on the quenching of ZrMOF nanoparticles, target-induced bioimaging is achieved upon binding with the target.
Biodetection
Since fluorescent nanomaterials can amplify the fluorescent signals significantly and be compatible with organisms, there is increasingly more research on their application in the rapid detection of biomolecules [117]. It will shorten the analysis time to a large extent if we are able to establish a real-time detection system by fluorescent nanomaterials. It has been discovered that multiple detection can be achieved by using QDs probes simultaneously [118, 119].
Pathogen Detection
Pathogens have been an unignorable threat to human health for centuries and these include many types of microorganisms ranging from bacteria (pathogenic Escherichia coli, Salmonella, and Streptococcus pneumoniae ) and viruses (Coronavirus, Influenza virus and hepatitis virus ). However, conventional methods for pathogen detection still need improvement of detection limits and detection speeds. For their applications in detecting pathogens, Tan and co-workers reported a bioconjugated nanoparticle-based biodetection for in situ pathogen quantification, which cost less than 20 min [120]. Tan’s success promises that quick and convenient pathogen detection is possible and can be achieved with these ingenious nanomaterials in the future. Here, we list diverse pathogens detected by fluorescent nanomaterials as shown in Table 2 [119, 121,122,123,124,125,126,127,128,129].
Nucleic Acid Detection
Apart from pathogen detection, fluorescent nanomaterials have also aroused more and more interest of scientists in the detection of DNA. Owing to the merits that a number of biomolecules can be attached to the surface of fluorescent nanomaterials, the signal intensity of fluorescent nanomaterials in DNA detection can be enhanced significantly. Tan and co-workers developed an DNA detection method to detect gene products using bioconjugated dye-doped fluorescent silica nanoparticle with high sensitivity and photostability [130]. Although the analysis of nucleic acid has been successfully achieved by real-time nanomaterial fluorescence systems, there are still many shortcomings such as complex procedures or expensive instruments. To address these disadvantages, Wang’s group introduced a highly sensitive and visualized detection of nucleic acid by the combination of strand exchange amplification (SEA) and lateral flow assay strip (LFA) [131]. The system, which is possible to be widely used in areas requiring limited resource, is mainly characterized by integrating SEA with LFA (Fig. 6). There is no denying that the extremely high fluorescent signal for bioanalysis plays an irreplaceable role in these applications.
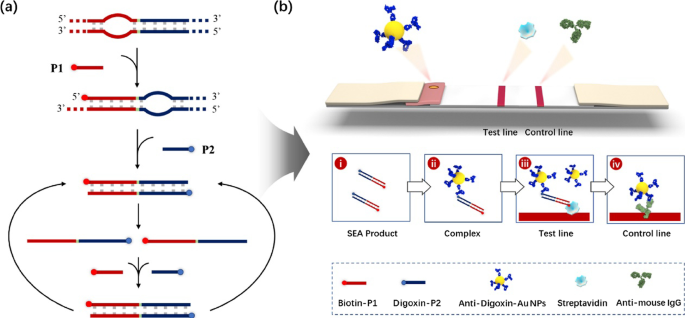
Schematic illustration of the SEA-LFA strip for the detection of nucleic acids [110]
Drug Detection
In the field of drug analysis, a facile and low-cost analytical method is always in demand for high-speed detection of specific pharmaceutical compounds. Real-time detection of drugs can be achieved with selective and sensitive fluorescent nanomaterials owing to their outstanding optical properties. In the past decade, the modification of nanomaterials has lowered their detection limit and improved their detection accuracy significantly. Recently, it is reported that ampicillin can be detected in serum sample based on aptamer, its complementary strand (CS) and gold nanoparticles (AuNPs) [132]. The limit of detection (LOD) of this method can be as low as 29.2 pM. However, there are still many limitations in the detection of drugs or target molecules in vivo. Due to low selectivity, conventional fluorescent nanomaterials inevitably generate false positive results and adverse effects in vivo. In addition, current tracking systems can hardly realize real-time tracking because of insufficient labels and excitation sources. Considering the above limitations, a new method using up/down conversion (UC/DC) PL nanomaterials has attracted increasing attention. Seo et al. reported a single-photon-driven UC/DC system which demonstrated outstanding performance in the detection of heavy metal ion (i.e. Hg 2+ ) in mussels [133]. LOD of the nanohybrids was ca. 1 nM. This system is appealing to researchers in the field of fluorescent nanomaterials for biomedical applications.
Drug Delivery
Until now, the technology of treating cancers with high efficiency and targeting function is not perfect enough. Under most circumstances, the anticancer drugs are distributed and released extensively in the body, which endangers the healthy cells and tissues irreversibly. Currently, a large variety of carriers for drug delivery have been designed. However, we can hardly supervise the distribution and result of the whole delivery process. Benefiting from the recent development of the surface modification technique, fluorescent materials capped with polymers like polyethylene glycol (PEG) can bond with drugs strongly and firmly. Then, the loaded drugs will be released in response to certain conditions such as pH, osmotic gradient and the surrounding environment. However, it should be confirmed whether the drugs are transported to the specific site or not. It’s also necessary for us to consider more details such as how much of the drugs is released in different positions. Aside from being drug carriers, fluorescent nanomaterials can also demonstrate the consequences of intracellular uptake due to their fluorescence property. QDs have been applied to monitor some important properties, such as delivery efficiency, release rate and distribution of drug molecules in vivo, which are beneficial for scientists in order to understand the specific targeting pathways of drug delivery within living cells. Duan and co-workers reported a facile pH-responsive fluorescent CDs drug delivery system [134]. Loaded with dox which is effective for gastric cancer, intracellular drug delivery and tracking could be simultaneously realized in patients (Fig. 7). The report highlighted the ability of fluorescent CDs to label and track the drug delivery process for at least 48 h, which showed a great potential in bioimaging, biolabeling and traceable drug delivery. Duan et al. designed a pH and receptor dual-responsive drug delivery system [135]. Hyaluronic acid was covalently attached to the surface of CDs, and doxorubicin was loaded by electrostatic self-assembly. In the tumor microenvironment (pH 5.6), the drug is released rapidly from the drug delivery system, while in the normal physiological environment (pH 7.4), the drug is hardly released. Endocytosis occurs when the drug delivery system reaches CD44 which is a receptor rich in tumor cells and can bind specifically to the hyaluronic acid. In addition, carbon nanotubes can be used for drug delivery by virtue of their high loading efficiency. Strong π-π interactions play a critical role in binding therapeutic agents with carbon nanotubes, which can be broke through changing external conditions, resulting in the release of drugs in specific position. Pennetta et al. functionalized single and multi-walled carbon nanotubes with a pyrrole derived compound to form a doxorubicin stacked drug delivery system. Biological studies showed that the synthesized nano-conveyors can effectively deliver the drug into cell lines and improve the therapeutic effects of doxorubicin [136].
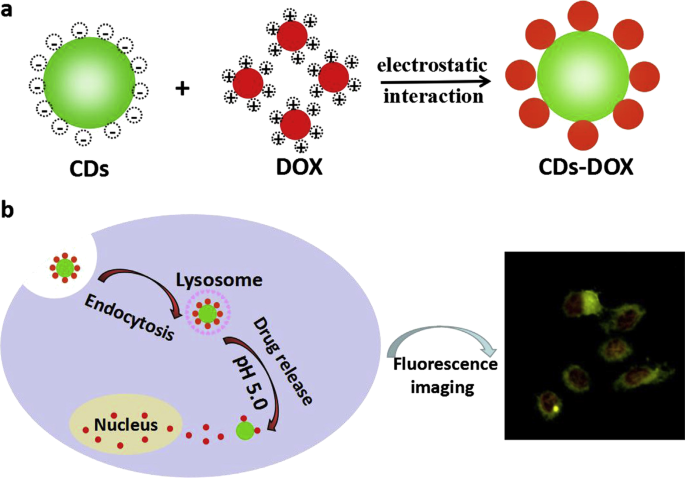
Schematic illustration of the preparation (a ) and cellular uptake (b ) of the CDs-DOX drug delivery system [113]
Photodynamic Therapy
Photodynamic therapy (PDT) is a novel therapy method for tumors which utilizes the interaction between light and photosensitizer. In PDT, reactive oxygen species (ROS) is produced from oxygen by photosensitizers in the condition of specific wavelengths of light (mostly in the area of near infrared light). The specific mechanism is presented in Fig. 8. ROS includes singlet oxygen, superoxide radicals, hydrogen peroxide, and hydroxyl radicals that possess strong cytotoxicity which cause significant destruction of tumor cells. However, there exist many defects such as limited penetration depth [137], hydrophobic properties [138], photobleaching [139], complicated procedure [140], and tumor hypoxia. PDT agents can hardly be dissolved and they will disperse extensively in vivo once they are taken, making it impossible to be targeted and selected. Fluorescent nanomaterial based photodynamic therapy developed fast in recent years [141]. Combined with the unique properties that QDs possess, such as high fluorescent efficiency and great spectral resolution, the effect of PDT can be enhanced. Barberi-Heyob, M and coworkers significantly enhanced the photodynamic efficiency with a concentration of 8 nM because of the light dose-dependent response [142]. In addition, photodynamic therapy can sometimes do harm to the skin and eyes of patients due to its photosensitive side-effect. To alleviate these adverse effects, a novel nanoparticle-based drug carrier for photodynamic therapy is reported which can provide stable aqueous dispersion of hydrophobic photosensitizers. Meanwhile, the key step of photogeneration of singlet oxygen was preserved, which is necessary for photodynamic action [143]. It is obvious that QDs combined photodynamic therapy will replace the conventional PDT someday.
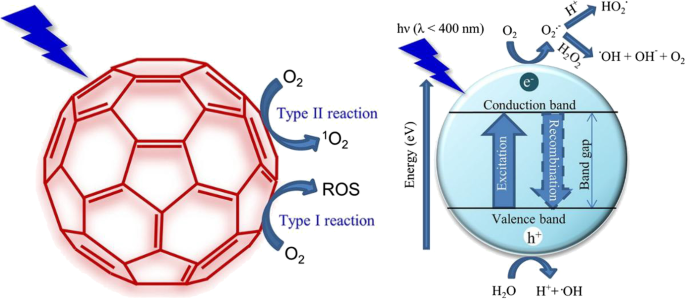
Schematic illustration of producing reactive oxygen species (ROS) for the photodynamic therapy (PDT) [119]
Challenges
Synthesis Challenges
Achieving Uniform Distribution
In the synthesis process, the diameter and size distributions of FNMs can be hardly distributed uniformly due to the agglomeration of small particles. This could be fatal to the optical properties of FNMs in biomedical application. For this reason, the applications of FNMs are still at the laboratory scale. It has been confirmed that the surface properties primarily determine the agglomeration state of the nanoparticles and their size. Therefore, surface modification is promising to achieve uniform distribution of FNMs by altering their surface properties [144]. To date, silanized QDs have been widely used because the polymerized silica coating increases the stability in buffers under physiological conditions [145]. Carbon dots synthesized by hydrothermal reaction using water-soluble base were reported to be difficult to control the size and distribution of grain boundary [146]. Khanam et al. reported a facile and novel synthetic method for the preparation of hydroxyl capped CDs using an organic base and a surfactant (Triton X-100) to modify the surface. A narrow particle size distribution at 7.2 nm was found in Raman and DLS studies, which is smaller than the majority of the particles falling within the range of below 10 nm in diameter [147].
Fluorescence Quantum Yield
Fluorescence quantum yield plays a crucial role for FNMs in their efficiency for on-demand light emission. Tunable and highly fluorescent CDs can be prepared with the surface functionalization approach. Nitrogen-doped FNMs are reported to have improved fluorescence quantum yield. With increasing nitrogen content, fluorescence quantum yield can be increased to as high as 56% at high synthesis temperature [148]. A facile strategy was also developed to tune the photoluminescent properties of CDs using a microwave irradiation, with citric acid and nitrogen-containing branched polyethyleneimine (b-PEI) as precursors. At intermediate levels of b-PEI, the CDs produced a high photoluminescence yield [149]. Lin et al. explored carbon dots with a high-fluorescence quantum yield rate synthesized from L-cysteine and citric acid by the microwave-assisted method. The obtained carbon dots exhibited a high-fluorescence quantum yield (up to 85%), which is due to the combination of amidogens and sulfydryl with carbon dots, and henceforth bringing the improved fluorescence property [150]. The above examples demonstrate that nitrogen or other electron-rich atoms like sulphur can obtain satisfying fluorescence quantum yield.
Aggregation-Caused Quenching
Fluorescent molecules can emit light with high efficiency in dilute solution. However, in concentrated solution or solid state, their fluorescence will be weakened or even disappear. This phenomenon is called Aggregation-Caused Quenching (ACQ) [151]. This problem has been puzzling scientists for almost 150 years, thus hindered the extensive application of fluorescent dyes.
In order to make effective use of fluorescent dyes, scientists have attempted many methods. Most of them focused on reducing the concentration of fluorescent dyes to prevent ACQ effect. Tang et al. discovered the phenomenon of Aggregation-Induced Emission (AIE) [152]. Based on rationally designed molecules, the fluorescence of organic molecules in solid state can be attained. Still, for more than one hundred thousand different fluorescent dyes in the world, the problem of ACQ has not been completely resolved. As long as they aggregate together, ACQ will make them lose their fluorescent properties.
It is almost impossible for high concentration or solid state FNMs to show reliable fluorescence due to fluorescence quenching. Although fluorescence quenching can be used as a sensitive signal to indicate substrate concentration in analytical chemistry, [153] in the most circumstances, however, fluorescence quenching is undesirable for FNMs because it always has considerable influence on bioimaging and biodetection. To overcome this long-standing problem, Benson et al. reported a universal solution with the discovery of a class of materials called small-molecule ionic isolation lattices (SMILES) [154]. SMILES are simple to make by mixing cationic dyes with anion-binding cyanostar macrocycles. We draw inspiration from their findings and believe that similar results can be obtained if we replace cationic dyes with cationic modified FNMs.
Application Challenges
Drawbacks of UV Light FNMs
Although FNMs realized the great-leap-forward from in vitro imaging to in vivo imaging, the emission fluorescence of most of FNMs is distributed in ultraviolet region or short wavelength visible region, which limits the optical imaging in living organisms. Moreover, use of UV light for monitoring living processes in cells and tissues has some potential drawbacks as long‐term irradiation of living cells may cause DNA damage and cell death. Therefore, the development of FNMs in near-infrared region is urgently needed in the future. Although NIR FNMs have deep tissue penetration, NIR detectors and filters are needed as the excitation and emission wavelengths are too close to each other, which restricts their range of application.
Interference in Biological Environment
Almost all biological tissues will produce significant autofluorescence under short wavelength, UV and visible light radiation [155]. Autofluorescence reduces the signal‐to‐background ratio and often interferes seriously with the visual effects. Some substances in the substrate of biological tissues also have great influence on the fluorescence, which reduces the selectivity of FNMs significantly. Until now, although the application of FNMs in mice showed acceptable outcomes, it is still difficult to achieve similar results in larger mammals. Much higher luminous efficiency under low power density excitation is required to avoid the background signal interference. Furthermore, temperature and pH conditions of the biological environments strongly affect the fluorescence of some substances as well. Therefore, satisfying fluorescence of FNMs at 37 °C and physiological pH should be guaranteed. It's worth noting that the pH in tumor is lower than normal tissues. Hence, fluorescence with high selectivity in acid environment will improve the efficiency of FNMs.
Biocompatibility
Biocompatibility refers to materials or systems that are nontoxic, safe and not causing physiological or immunological reactions. QDs with unique quantum confinement effect and electro-optical properties are attractive for biomedical applications. However, toxic effects of traditional semiconductor QDs made of heavy metal ions have serious safety concerns for their undesired environmental or health effects. In the purpose of circumventing this problem, core–shell structure modification of QDs by using biocompatible ligands or polymers is one way to effectively minimize toxic effects of traditional QDs. Furthermore, scientists are searching for heavy metal-free QDs formulations. Non-toxic or less toxic carbon dots and silica nanoparticles have shown their potential as the ideal FNMs for biomedical applications. Impurities brought in the process of syntheses may influence the biocompatibility of fluorescent nanomaterials. In order to reduce the influence of toxic impurities, green synthesis methods have been arousing the interest in biomedical fields. Chowdhury et al. utilized cacao extract which is a natural product as a reducing and stabilizing agent in the synthesis of gold nanoparticles [156]. Oxalic acid, as a constituent of cacao, can reduce Au 3+ in HAuCl4 to metallic gold and stabilize the resultant nanoparticle colloidal solution. In vitro studies suggested that the cacao derived gold nanoparticles are biocompatible and suitable for biomedical applications. For MOFs, appropriate metal ions and ligands must be selected to lower the toxicity. Wang et al. employed Fe, Ti and Zr as constituents of MOFs, which are harmless and even beneficial elements to the body [157]. In vitro studies indicate that the proposed material has good biocompatibility and safety in biomedical application. What’s more, it is necessary to consider whether the difference in composition, surface charge, or modified group will have different biological effects. Taking these factors into account, we can improve the biocompatibility of FNMs with rational design.
Conclusions
Benefiting from the unique properties of fluorescent nanomaterials, some limitations and barriers of conventional materials and methods in biomedical applications can be broken through. In this review, we comprehensively present the synthesis methods and applications of fluorescent nanomaterials. The advanced synthesis methods can offer us the fluorescent nanomaterials with ideal morphology, size ranges and structures. Meanwhile, the more convenient syntheses can lower the manufacturing cost of fluorescent nanomaterials, which is critical to their widespread applications in biomedical fields. Based on the improved synthesis techniques, the performance of fluorescent nanomaterials is bound to leap in their applications. With the development of fluorescent nanomaterials, bioimaging, biodection, drug delivery and photodynamic therapy will be more widely applied in the diagnosis and treatment of diseases. Finally, challenges in synthesis and biomedical applications point out exiting questions and developing direction. We hope that this review can bring some new insights to the development of fluorescent nanomaterials.
Availability of data and materials
All data and materials are available without restrictions.
Abbreviations
- FNMs:
-
Fluorescent nanomaterials
- PL:
-
Photoluminescence
- CDs:
-
Carbon dots
- QDs:
-
Quantum dots
- b-PEI:
-
Branched polyethyleneimine
- SWCNTs:
-
Single-walled carbon nanotubes
- CQDs:
-
Carbon quantum dots
- GQDs:
-
Graphene quantum dots
- NCQDs:
-
Nitrogen-doped carbon quantum dots
- NSCDs:
-
Nitrogen and sulfur doped carbon dots
- QY:
-
Quantum yield
- MCDs:
-
Multi-doped carbon dots
- MWCHTs:
-
Multi-walled carbon nanotubes
- GO:
-
Graphene oxide
- rGO:
-
Reduced graphene oxide
- CVD:
-
Chemical vapor deposition
- AuNPs:
-
Gold nanoparticles
- Ag NCs:
-
Ag nanoclusters
- Cu NCs:
-
Cu nanoclusters
- NPs:
-
Nanoparticles
- TEOS:
-
Tetraethylorthosilicate
- MR:
-
Magnetic resonance
- COFs:
-
Covalent-organic frameworks
- TNP:
-
2,4,6-Trinitrophenol
- MOFs:
-
Metal organic frameworks
- ACQ:
-
Aggregation-caused quenching
- NIR:
-
Near infrared
- SEA:
-
Strand exchange amplification
- LFA:
-
Lateral flow assay strip
- CS:
-
Complementary strand
- LOD:
-
Limit of detection
- UC:
-
Upconversion
- DC:
-
Downconversion
- PDT:
-
Photodynamic therapy
- ROS:
-
Reactive oxygen species
- AIE:
-
Aggregation-induced emission
- SMILES:
-
Small-molecule ionic isolation lattices
Nanomatériaux
- Capteurs et processeurs convergent pour les applications industrielles
- Quelle est la prochaine étape pour l'impression 3D – Applications biomédicales
- Les cinq principaux problèmes et défis de la 5G
- Nanoparticules pour le traitement du cancer :progrès actuels et défis
- Dernières avancées et applications de la technologie IoT
- EconCore octroie une licence à Fynotej pour les applications automobiles NA et les avancées dans les thermoplastiques hautes performances (HPT)
- Variateurs pour applications de réfrigération et de refroidissement industriels
- Tours oscillants pour applications d'alésage et de tournage
- Comprendre les avantages et les défis de la fabrication hybride