Une nouvelle conception d'un système intelligent d'administration de médicaments basé sur des particules de nano-antenne
Résumé
Le système d'administration de médicament composé de nanoparticules joue un rôle important dans l'interaction avec les ganglions lymphatiques. Il existe trois principaux types de lymphocytes :les lymphocytes B, les lymphocytes T et les cellules tueuses naturelles. Lorsque les cellules du système immunitaire deviennent cancérigènes, elles agressent les cellules du corps. Le liquide lymphatique joue un rôle important dans l'attaque des cellules saines du corps; par conséquent, cet article visait à concevoir un système d'administration de médicaments, qui peut diriger efficacement les nanoparticules pour cibler les cellules infectées, contribuant ainsi à l'élimination à grande vitesse de ces cellules. La conception proposée dépend de l'interaction entre ces molécules, et le nano-contrôleur intelligent a la capacité de guider les nanoparticules par contact anaérobie. La conception proposée a prouvé que plus la taille et la densité des nanoparticules sont petites, moins la viscosité dynamique du liquide serait, ce qui refléterait sa résistance à l'écoulement. En outre, il a été conclu que les molécules d'hydrogène jouent un rôle important dans la réduction de la résistance au liquide lymphatique en raison de leur faible densité.
Introduction
Les options actuelles de traitement du cancer comprennent la chirurgie, la radiothérapie et la chimiothérapie. Ces stratégies de traitement endommagent également les tissus ordinaires et entraînent l'annihilation partielle de la croissance maligne. Par conséquent, la nanotechnologie peut surmonter ces lacunes en ciblant spécifiquement les cellules nocives et les néoplasmes, en réséquant directement les tumeurs et en augmentant l'efficacité des modalités de traitement à base de rayonnement et d'autres. Cela peut réduire considérablement les effets indésirables du traitement et augmenter le taux de survie. La nanotechnologie est un outil prometteur pour le traitement de la croissance maligne car elle offre des modalités de traitement plus récentes et meilleures grâce à l'utilisation de nanomatériaux. Les nanoparticules peuvent cibler spécifiquement de nombreuses molécules exprimées de manière différentielle sur les cellules cancéreuses. La région généralement vaste du profil aérodynamique des nanoparticules peut être fonctionnalisée avec des ligands tels que des petites particules et des anticorps peptidiques à chaîne corrosive désoxyribonucléique ou corrosive ribonucléique. Les ligands sont utilisés comme médicament et dans des applications théranostiques. Les propriétés physiques des nanoparticules, telles que la distraction vitale et la reradiation, peuvent également être utilisées pour affecter les tissus malades, comme dans les applications d'élimination au laser et d'hyperthermie [1].
Le logiciel innovant de nanoparticules et l'élément pharmaceutique actif permettront également l'exploration d'un répertoire plus large d'ingrédients actifs. Par conséquent, la cargaison immunogène et le revêtement de surface sont étudiés en tant qu'adjuvants à la chimiothérapie traditionnelle et à l'aide de nanoparticules. Cette stratégie innovante comprend la conception de nanoparticules en tant qu'antigène artificiel présentant sur les cellules et des dépôts in vivo de facteurs de stimulation qui exercent des effets anti-tumoraux. La nanotechnologie représente un domaine de recherche actif avec de nombreuses applications. Les nanoparticules ont suscité un intérêt dans la technologie médicale en raison de leurs caractéristiques physico-chimiques ajustables telles que le point d'indice de décongélation, l'hydrophilie, la conduction électrique et thermique, l'activité catalytique, l'absorption de la lumière et la diffusion [2]. En principe, les nanomatériaux sont décrits comme des matériaux avec des particules dans la gamme de 1 à 100 nm. Il existe plusieurs textes législatifs dans l'Union européenne et aux États-Unis faisant spécifiquement référence à la recherche médicale utilisant des nanomatériaux. Cependant, il n'existe pas de définition internationalement acceptée des nanomatériaux. Différentes organisations considèrent différents concepts de nanomatériaux [3]. L'un des objectifs du système d'administration de médicaments à base de nanoparticules est de traiter le liquide lymphatique avec des cellules cancéreuses. Un système d'administration de médicament composé de nanoparticules en interaction avec les ganglions lymphatiques est illustré à la figure 1.
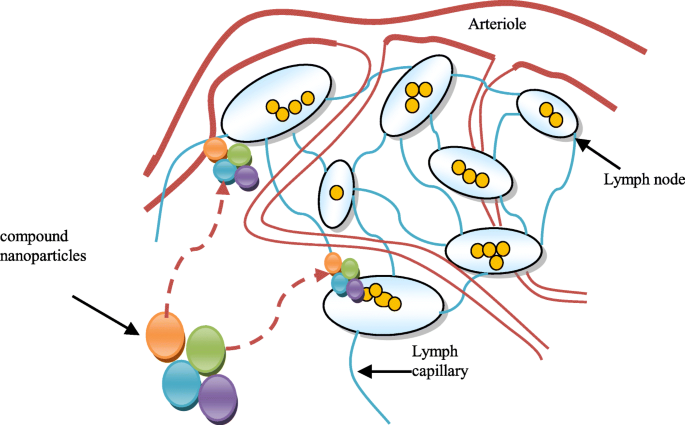
Système d'administration de médicament composé de nanoparticules et son interaction avec les ganglions lymphatiques
La Food and Drug Administration des États-Unis fait allusion aux nanomatériaux en tant que matériaux avec des particules de l'ordre de 1 à 100 avec des propriétés différentes de celles du matériau en vrac [4, 5]. Des nanofibres, des nanoplaques, des nanofils, des points quantiques et d'autres matériaux connexes ont été caractérisés [6]. Les nanoparticules lipidiques solides (SLN) sont un type de nanoparticules lipidiques (LN), qui peuvent être construites en utilisant des lipides solides [7]. Des versions ultérieures de SLN ont été développées, telles que les transporteurs lipidiques nanostructurés (NLC), qui représentent la deuxième ère de LN [8]. Le SLN et le NLC sont tous deux construits à partir de lipides solides. La structure intérieure du SLN contient des lipides solides, tandis que le NLC est développé en utilisant un mélange de lipides solides et liquides, qui produisent une section transversale de pierre précieuse [9, 10]. Ces défauts ont en outre été rapportés pour les GLS à la lumière du fait que les GLS qui contiennent de nombreux segments lipidiques solides peuvent être utilisés dans des applications médicales [11, 12]. Les nanoparticules polymères (PN) peuvent être construites à partir de polymères naturels ou de matériaux inorganiques, par exemple la silice [13]. Les polymères ou lipides forment le noyau des NP, qui améliorent la stabilité et l'administration du médicament et offrent une forme et une taille uniformes [14]. Le PN peut être décrit comme des nanocapsules ou des nanosphères. Les nanocapsules contiennent de l'huile dans une structure vésiculaire avec un médicament [15, 16], tandis que les nanosphères contiennent des chaînes polymères sans huile [17, 18]. Un médicament est emballé dans des PN par mélange avec le polymère. L'incorporation du médicament est assurée dans les nanoparticules au moment de la polymérisation. Les PN sont chargés d'un médicament en le dissolvant, en le dispersant ou en l'adsorbant artificiellement dans les constituants du réseau polymère [19, 20]. Il existe trois types de lymphocytes :les lymphocytes B, les lymphocytes T et les cellules tueuses naturelles. Les cellules B fabriquent des anticorps qui attaquent les micro-organismes envahisseurs, tandis qu'elles attaquent également le système immunitaire lorsqu'elles deviennent cancérigènes. Par conséquent, compte tenu du rôle important du liquide lymphatique dans l'auto-immunité, l'objectif de cet article était de concevoir un système intelligent d'administration de médicaments basé sur des particules de nanoantenne. Ainsi, le système contient de nombreuses nanoparticules en différentes quantités. La section suivante présente la conception d'un système intelligent d'administration de médicaments.
Conception d'un système d'administration de médicaments nano-intelligent
Le système d'administration de médicament nano-intelligent proposé contient un nanocontrôleur actionné par une source électrique de nanoparticules constituées d'un matériau nano-piézoélectrique. Le référentiel complexe de nanoparticules comporte un certain nombre de micro-dépôts. Chaque petit référentiel contient un type de nanoparticules. Une molécule de nanoparticule contient une nanoantenne conçue pour communiquer avec le nanocontrôleur. Le système d'administration de médicaments nano-intelligent proposé contient également des nanotubes de carbone pour l'administration rapide de médicaments aux cellules cancéreuses. Il peut être associé aux cellules infectées, comme le montre la figure 2. Le système commence par envoyer des nanoparticules aux cellules cancéreuses appelées « nanoparticules exploratoires ». Ces molécules, au moyen de la communication anaérobie, envoient au nanocontrôleur l'image complète de leur position à l'intérieur des cellules. Sur la base de la situation rencontrée par les nanoparticules exploratoires, le nano-contrôleur envoie des nanoparticules de nombre, de type et de densité différents aux cellules cancéreuses, sur la base des informations collectées à partir des nanoparticules exploratoires. Ces nanoparticules sont appelées « nanoparticules de combat ».
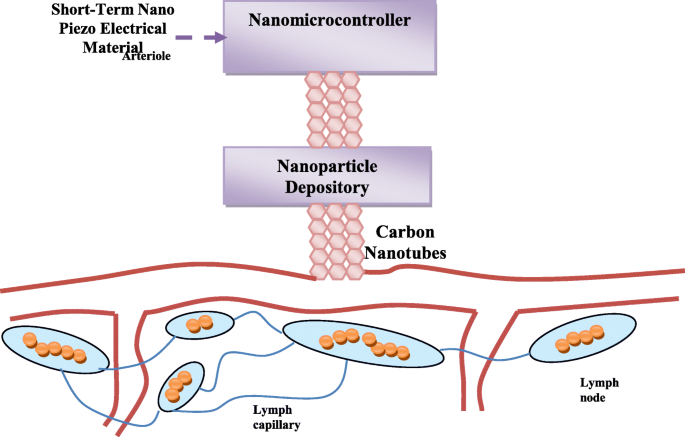
Structure générale montrant l'association du système médicamenteux proposé avec les cellules infectées
Ce n'est pas un processus aléatoire mais contrôlé par le nano-contrôleur en tenant compte de plusieurs aspects et logarithmes, ce qui assurera une livraison efficace et rapide des nanoparticules. Afin de livrer avec précision et rapidité les nanoparticules aux cellules cancéreuses, l'algorithme de recherche binaire compressif sera utilisé [21]. De plus, les nanoparticules seront délivrées dans différentes densités afin que le médicament devienne plus efficace. Ces méthodologies et leur mode opératoire en utilisant le nano-contrôleur sont illustrés sur la Fig. 3. La structure physique du nano-contrôleur est similaire à celle des nanoparticules, mais il est sous forme de métal afin qu'il puisse gagner de l'énergie électrique. pendant une courte période tout en travaillant. Ce métal contient une antenne sans fil ainsi qu'une petite mémoire qui contient les codes de fonctionnement avec un lien nanoparticulaire entre le nano-contrôleur et le magasin de nanoparticules. Le référentiel de nanoparticules contient plusieurs types différents de nanoparticules. L'ouverture et la fermeture, ainsi que la durée d'ouverture de la nano-porte, seront contrôlées pour adapter le nombre de particules à délivrer.
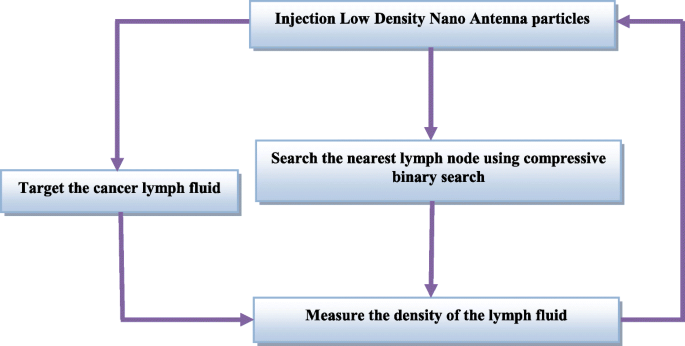
Processus d'envoi des nanoparticules combattantes aux cellules cancéreuses
Une description de la nature des nanoparticules utilisées dans le système pharmaceutique proposé
Dans la section suivante, la nature des nanoparticules utilisées dans le système d'administration de médicament proposé est discutée. Dans ce travail, des nanoparticules anaérobies de faible densité ont été utilisées comme décrit dans un rapport précédent [22].
Nanoparticules à faible densité
Considérez le processus d'administration de médicament des nanoparticules composées pour le cancer comme un processus de pénétration dans le liquide lymphatique, où une tumeur est entourée par le liquide lymphatique. La composition du mélanome ressemble au liquide lymphatique. Le modèle analytique proposé est basé sur un système de nanotubes constitué de trois types différents de nanoparticules. Les nanoparticules sont placées dans un liquide lymphatique de haute densité. Nous pouvons définir des nanoparticules spécifiques du solide A dans les coordonnées polaires sphériques comme A = (ra, a , φa), où ra est la coordonnée radiale de la nanoparticule du solide A, ϑa est la coordonnée zénithale de la nanoparticule du solide A, et φa est la coordonnée azimutale de la nanoparticule du solide A. Les coordonnées correspondantes du solide B sont B = (rb, ϑb, φb), respectivement, et les coordonnées correspondantes du solide N sont N = (rn, ϑn, φn), respectivement. Considérez qu'il existe deux propriétés du ganglion lymphatique, à savoir, tendre et enflé, qui sont affectées par les cellules cancéreuses du lymphome de Hodgkin. Le ganglion lymphatique avec la propriété tendre Tp peut être décrit comme Tp (N ,t ); cela signifie que la valeur de Tp en association avec le fluide de nanoparticules de N solide varie avec le temps. Considérons maintenant que l'effet total des nanoparticules composées dans la propriété tendre est défini comme :
$$ \mathrm{Tpt}=\mathrm{Tp}\ \left(A,t\right)+\mathrm{Tp}\ \left(B,t\right)+.\dots \dots \dots \dots + \mathrm{Tp}\ \left(N,t\right) $$ (1)Considérons le même cas pour la propriété gonflée, qui pourrait être définie comme :
$$ \mathrm{Tst}=\mathrm{Ts}\ \left(A,t\right)+\mathrm{Ts}\ \left(B,t\right)+.\dots \dots \dots \dots + \mathrm{Ts}\ \left(N,t\right) $$ (2)À partir des équations. 1 et 2, le taux de changement des deux propriétés avec le temps peut être déterminé comme :
$$ \frac{\partial \left(\mathrm{Tp}\left(A,t\right)\right)}{\partial t}+\frac{\partial \left(\mathrm{Tp}\left( B,t\right)\right)}{\partial t}+\dots \frac{\partial \left(\mathrm{Tp}\left(N,t\right)\right)}{\partial t}=\frac{\mathrm{\partial Tp}(t)}{\mathrm{\partial t}} $$ (3) $$ \frac{\partial \left(\mathrm{Ts}\left(A,t\ right)\right)}{\partial t}+\frac{\partial \left(\mathrm{Ts}\left(B,t\right)\right)}{\partial t}+\dots \frac{\ partiel \left(\mathrm{Ts}\left(N,t\right)\right)}{\partial t}=\frac{\mathrm{\partial Ts}(t)}{\partial t} $$ ( 4)Le point dans le liquide lymphatique qui peut être occupé par une nanoparticule de N solide est défini comme :
$$ {\mathrm{Po}}_n=\mathrm{Po}{\left(\mathrm{po},t\right)}_n $$ (5)Considérons qu'une nanoparticule du dérivé N solide du liquide lymphatique tendre est définie comme \( \frac{\partial {\left(\mathrm{Tp}\left(N,t\right)\right)}_{\ mathrm{po}}}{\partial t} \), alors le dérivé matériel composé du liquide lymphatique tendre serait égal à :
$$ \frac{\partial {\left(\mathrm{Tp}\left(A,t\right)\right)}_{\mathrm{po}}}{\partial t}+\frac{\partial { \left(\mathrm{Tp}\left(B,t\right)\right)}_{\mathrm{po}}}{\partial t}+\dots \frac{\partial {\left(\mathrm{ Tp}\left(N,t\right)\right)}_{\mathrm{po}}}{\partial t}=\frac{\mathrm{\partial Tp}{(t)}_{\mathrm{ po}}}{\partial t} $$ (6) $$ \frac{\partial {\left(\mathrm{Ts}\left(A,t\right)\right)}_{\mathrm{po} }}{\partial t}+\frac{\partial {\left(\mathrm{Ts}\left(B,t\right)\right)}_{\mathrm{po}}}{\partial t}+ \dots \frac{\partial {\left(\mathrm{Ts}\left(N,t\right)\right)}_{\mathrm{po}}}{\partial t}=\frac{\mathrm{ \partial Ts}{(t)}_{\mathrm{po}}}{\partial t} $$ (7)Les composantes de vitesse correspondantes du solide N sont prises comme (v rn , v ϑn , v φn ). Ensuite, la vitesse d'écoulement des particules de N solide est représentée à l'aide des équations de Navier-Stokes à la viscosité dynamique dν du liquide lymphatique, et p est la pression et ρ est la densité du liquide lymphatique comme suit :
$$ \frac{\partial {v}_{\mathrm{rn}}}{\partial t}+{v}_{\mathrm{rn}}\frac{\partial {v}_{\mathrm{rn }}}{\mathrm{\partial rn}}+\frac{v_{\upvartheta \mathrm{n}}}{\mathrm{rn}}\frac{\partial {v}_{\mathrm{rn}} }{\mathrm{\partial \upvartheta n}}+\frac{v_{\upvarphi \mathrm{n}}}{\mathrm{rn}\ \mathrm{sin}\upvartheta \mathrm{n}}\frac{ \partial {v}_{\mathrm{rn}}}{\mathrm{\partial \upvarphi n}}-\frac{v_{\upvartheta \mathrm{n}}^2}{\mathrm{rn}}- \frac{v_{\upvarphi \mathrm{n}}^2}{\mathrm{rn}}+\frac{1}{\rho}\frac{\partial p}{\mathrm{\partial rn}}- \mathrm{d}\upnu \left[\frac{1}{{\mathrm{rn}}^2}\frac{\partial }{\mathrm{\partial rn}}\left({\mathrm{rn} }^2\frac{\partial {v}_{\mathrm{rn}}}{\mathrm{\partial rn}}\right)+\frac{1}{{\mathrm{rn}}^2\mathrm {sin}\upvartheta \mathrm{n}}\frac{\partial }{\mathrm{\partial \upvartheta n}}\left(\mathrm{sin}\upvartheta \mathrm{n}\frac{\partial {v }_{\mathrm{rn}}}{\mathrm{\partial \upvartheta n}}\right)+\frac{1}{{\mathrm{rn}}^2{\sin}^2\upvartheta \mathrm {n}}\frac{\partial^2{v}_{\ma thrm{rn}}}{\partial {\upvarphi \mathrm{n}}^2}+-\frac{2{v}_{\mathrm{rn}}}{{\mathrm{rn}}^2} -\frac{2}{{\mathrm{rn}}^2\sin \upvartheta \mathrm{n}}\frac{\partial \left({v}_{\upvartheta \mathrm{n}}\mathrm{ sin}\upvartheta \mathrm{n}\right)}{\mathrm{\partial \upvartheta n}}-\frac{2}{{\mathrm{rn}}^2\mathrm{sin}\upvartheta \mathrm{ n}}\frac{\partial {v}_{\upvarphi \mathrm{n}}}{\partial_{\upvarphi \mathrm{n}}}\right]=0 $$ (8)La viscosité dynamique dν du liquide lymphatique est calculée comme suit :
$$ \mathrm{d}\upnu =\frac{\left[\frac{\partial {v}_{\mathrm{rn}}}{\partial t}+{v}_{\mathrm{rn}} \frac{\partial {v}_{\mathrm{rn}}}{\mathrm{\partial rn}}+\frac{v_{\upvartheta \mathrm{n}}\partial {v}_{\mathrm{ rn}}}{\mathrm{rn}\ \mathrm{\partial \upvartheta n}}+\frac{v_{\upvarphi \mathrm{n}}}{\mathrm{rn}\ \mathrm{sin}\upvartheta \mathrm{n}}\frac{\partial {v}_{\mathrm{rn}}}{\mathrm{\partial \upvarphi n}}-\frac{v_{\upvartheta \mathrm{n}}^2 }{\mathrm{rn}}-\frac{v_{\upvarphi \mathrm{n}}^2}{\mathrm{rn}}+\frac{1\ \partial p}{\uprho \partial \mathrm{ rn}}\right]}{\left[\frac{1}{{\mathrm{rn}}^2}\frac{\partial }{\mathrm{\partial rn}}\left({\mathrm{rn }}^2\frac{\partial {v}_{\mathrm{rn}}}{\mathrm{\partial rn}}\right)+\frac{1}{{\mathrm{rn}}^2\ mathrm{sin}\upvartheta \mathrm{n}}\frac{\partial }{\mathrm{\partial \upvartheta n}}\left(\mathrm{sin}\upvartheta \mathrm{n}\frac{\partial { v}_{\mathrm{rn}}}{\mathrm{\partial \upvartheta n}}\right)+\frac{1}{{\mathrm{rn}}^2{\sin}^2\upvartheta \ mathrm{n}}\frac{\partiel ^2{v}_{\mathrm{rn}}}{\partial {\upvarphi \mathrm{n}}^2}+-\frac{2{v}_{\mathrm{rn}}}{{\ mathrm{rn}}^2}-\frac{2}{{\mathrm{rn}}^2\sin \upvartheta \mathrm{n}}\frac{\partial \left({v}_{\upvartheta \ mathrm{n}}\mathrm{sin}\upvartheta \mathrm{n}\right)}{\mathrm{\partial \upvartheta n}}-\frac{2}{{\mathrm{rn}}^2\mathrm {sin}\upvartheta \mathrm{n}}\frac{\partial {v}_{\upvarphi \mathrm{n}}}{\partial_{\upvarphi \mathrm{n}}}\right]} $$ ( 9)Les équations de Navier-Stokes des solides A et B pourraient être représentées par des équations. 8 et 9. Ainsi, l'Éq. 9 pourrait être représenté comme suit :
$$ \mathrm{d}\upnu =\frac{\left[\frac{\partial {v}_{\mathrm{rn}}}{\partial t}+{v}_{\mathrm{rn}} \frac{\partial {v}_{\mathrm{rn}}}{\mathrm{\partial rn}}+\frac{v_{\upvartheta \mathrm{n}}}{\mathrm{rn}}\frac {\partial {v}_{\mathrm{rn}}}{\mathrm{\partial \upvartheta n}}+\frac{v_{\upvarphi \mathrm{n}}}{\mathrm{rn}\ \mathrm {sin}\upvartheta \mathrm{n}}\frac{\partial {v}_{\mathrm{rn}}}{\mathrm{\partial \upvarphi n}}-\frac{v_{\upvartheta \mathrm{ n}}^2}{\mathrm{rn}}-\frac{v_{\upvarphi \mathrm{n}}^2}{\mathrm{rn}}+\frac{1}{\rho}\frac{ \partial p}{\mathrm{\partial rn}}\right]}{\left[\frac{1}{{\mathrm{rn}}^2}\frac{\partial }{\mathrm{\partial rn }}\left({\mathrm{rn}}^2\frac{\partial {v}_{\mathrm{rn}}}{\mathrm{\partial rn}}\right)+\frac{1}{ {\mathrm{rn}}^2\mathrm{sin}\upvartheta \mathrm{n}}\frac{\partial }{\mathrm{\partial \upvartheta n}}\left(\mathrm{sin}\upvartheta \ mathrm{n}\frac{\partial {\mathrm{v}}_{\mathrm{rn}}}{\mathrm{\partial \upvartheta n}}\right)+\frac{1}{{\mathrm{ rn}}^2{\sin}^2\upvartheta \math rm{n}}\frac{\partial^2{\mathrm{v}}_{\mathrm{rn}}}{\partial {\upvarphi \mathrm{n}}^2}-\frac{2{\ mathrm{v}}_{\mathrm{rn}}}{{\mathrm{rn}}^2}-\frac{2}{{\mathrm{rn}}^2\sin \upvartheta \mathrm{n} }\frac{\partial \left({\mathrm{v}}_{\upvartheta \mathrm{n}}\mathrm{sin}\upvartheta \mathrm{n}\right)}{\mathrm{\partial \upvartheta n}}-\frac{2}{{\mathrm{rn}}^2\mathrm{sin}\upvartheta \mathrm{n}}\frac{\partial {\mathrm{v}}_{\upvarphi \mathrm {n}}}{\partial_{\upvarphi \mathrm{n}}}\right]}=\frac{\left[\frac{\partial {\mathrm{v}}_{\mathrm{ra}}} {\mathrm{\partial t}}+{\mathrm{v}}_{\mathrm{ra}}\frac{\partial {\mathrm{v}}_{\mathrm{ra}}}{\mathrm{ \partial ra}}+\frac{{\mathrm{v}}_{\upvartheta \mathrm{a}}}{\mathrm{ra}}\frac{\partial {\mathrm{v}}_{\mathrm {ra}}}{\mathrm{\partial \upvartheta a}}+\frac{{\mathrm{v}}_{\upvarphi \mathrm{a}}}{\mathrm{ra}\ \mathrm{sin} \upvartheta \mathrm{a}}\frac{\partial {\mathrm{v}}_{\mathrm{ra}}}{\mathrm{\partial \upvarphi a}}-\frac{{\mathrm{v} }_{\upvartheta \mathrm{a}}^2}{\mathrm{ra}}- \frac{{\mathrm{v}}_{\upvarphi \mathrm{a}}^2}{\mathrm{ra}}+\frac{1}{\uprho}\frac{\mathrm{\partial p} }{\mathrm{\partial ra}}\right]}{\left[\frac{1}{{\mathrm{ra}}^2}\frac{\partial }{\mathrm{\partial ra}}\ gauche({\mathrm{ra}}^2\frac{\partial {v}_{\mathrm{ra}}}{\mathrm{\partial ra}}\right)+\frac{1}{{\mathrm {ra}}^2\mathrm{sin}\upvartheta \mathrm{a}}\frac{\partial }{\mathrm{\partial \upvartheta a}}\left(\mathrm{sin}\upvartheta \mathrm{a }\frac{\partial {v}_{\mathrm{ra}}}{\mathrm{\partial \upvartheta a}}\right)+\frac{1}{{\mathrm{ra}}^2{\ sin}^2\upvartheta \mathrm{a}}\frac{\partial^2{v}_{\mathrm{ra}}}{\partial {\upvarphi \mathrm{a}}^2}-\frac{ 2{v}_{\mathrm{ra}}}{{\mathrm{ra}}^2}-\frac{2}{{\mathrm{ra}}^2\sin \upvartheta \mathrm{a}} \frac{\partial \left({v}_{\upvartheta \mathrm{a}}\mathrm{sin}\upvartheta \mathrm{a}\right)}{\mathrm{\partial \upvartheta a}}-\ frac{2}{{\mathrm{ra}}^2\mathrm{sin}\upvartheta \mathrm{a}}\frac{\partial {v}_{\upvarphi \mathrm{a}}}{\partial_{ \upvarphi \mathrm{a}}}\right]}=\frac{ \left[\frac{\partial {v}_{\mathrm{rb}}}{\partial t}+{v}_{\mathrm{rb}}\frac{\partial {v}_{\mathrm{ rb}}}{\mathrm{\partial rb}}+\frac{v_{\upvartheta \mathrm{b}}}{\mathrm{rb}}\frac{\partial {v}_{\mathrm{rb} }}{\mathrm{\partial \upvartheta b}}+\frac{v_{\upvarphi \mathrm{b}}}{\mathrm{rb}\ \mathrm{sin}\upvartheta \mathrm{b}}\frac {\partial {v}_{\mathrm{rb}}}{\mathrm{\partial \upvarphi b}}-\frac{v_{\upvartheta \mathrm{b}}^2}{\mathrm{rb}} -\frac{v_{\upvarphi \mathrm{b}}^2}{\mathrm{rb}}+\frac{1}{\rho}\frac{\partial p}{\mathrm{\partial rb}} \right]}{\left[\frac{1}{{\mathrm{rb}}^2}\frac{\partial }{\mathrm{\partial rb}}\left({\mathrm{rb}}^ 2\frac{\partial {v}_{\mathrm{rb}}}{\mathrm{\partial rb}}\right)+\frac{1}{{\mathrm{rb}}^2\mathrm{sin }\upvartheta \mathrm{b}}\frac{\partial }{\mathrm{\partial \upvartheta b}}\left(\mathrm{sin}\upvartheta \mathrm{b}\frac{\partial {v}_ {\mathrm{rb}}}{\mathrm{\partial \upvartheta b}}\right)+\frac{1}{{\mathrm{rb}}^2{\sin}^2\upvartheta \mathrm{b }}\frac{\partial^2{v}_{\mathrm{ rb}}}{\partial {\upvarphi \mathrm{b}}^2}-\frac{2{v}_{\mathrm{rb}}}{{\mathrm{rb}}^2}-\frac {2}{{\mathrm{rb}}^2\sin \upvartheta \mathrm{b}}\frac{\partial \left({v}_{\upvartheta \mathrm{b}}\mathrm{sin}\ upvartheta \mathrm{b}\right)}{\mathrm{\partial \upvartheta b}}-\frac{2}{{\mathrm{rb}}^2\mathrm{sin}\upvartheta \mathrm{b}} \frac{\partial {v}_{\upvarphi \mathrm{b}}}{\partial_{\upvarphi \mathrm{b}}}\right]} $$ (10)Les particules sont en nano-dimensions; ainsi, leurs rayons seraient très petits, et pour simplifier, l'Eq. 10 est représenté comme suit :
$$ \mathrm{d}\upnu =\left[\frac{v_{\upvartheta \mathrm{n}}}{\mathrm{rn}}\frac{\partial {v}_{\mathrm{rn}} }{\mathrm{\partial \upvartheta n}}+\frac{v_{\upvarphi \mathrm{n}}}{\mathrm{rn}\ \mathrm{sin}\upvartheta \mathrm{n}}\frac{ \partial {v}_{\mathrm{rn}}}{\mathrm{\partial \upvarphi n}}-\frac{v_{\upvartheta \mathrm{n}}^2}{\mathrm{rn}}- \frac{v_{\upvarphi \mathrm{n}}^2}{\mathrm{rn}}+\frac{1}{\rho}\frac{\partial p}{\mathrm{\partial rn}}\ right]/\left[\frac{1}{{\mathrm{rn}}^2}\frac{\partial }{\mathrm{\partial rn}}\left({\mathrm{rn}}^2\ frac{\partial {v}_{\mathrm{rn}}}{\mathrm{\partial rn}}\right)+\frac{1}{{\mathrm{rn}}^2\mathrm{sin}\ upvartheta \mathrm{n}}\frac{\partial }{\mathrm{\partial \upvartheta n}}\left(\mathrm{sin}\upvartheta \mathrm{n}\frac{\partial {\mathrm{v} }_{\mathrm{rn}}}{\mathrm{\partial \upvartheta n}}\right)+\frac{1}{{\mathrm{rn}}^2{\sin}^2\upvartheta \mathrm {n}}\frac{\partial^2{\mathrm{v}}_{\mathrm{rn}}}{\partial {\upvarphi \mathrm{n}}^2}-\frac{2{\mathrm {v}}_{\mathrm{rn}}}{{\math rm{rn}}^2}-\frac{2}{{\mathrm{rn}}^2\sin \upvartheta \mathrm{n}}\frac{\partial \left({\mathrm{v}}_ {\upvartheta \mathrm{n}}\mathrm{sin}\upvartheta \mathrm{n}\right)}{\mathrm{\partial \upvartheta n}}-\frac{2}{{\mathrm{rn}} ^2\mathrm{sin}\upvartheta \mathrm{n}}\frac{\partial {\mathrm{v}}_{\upvarphi \mathrm{n}}}{\partial_{\upvarphi \mathrm{n}} }\right]=\left[\frac{{\mathrm{v}}_{\upvartheta \mathrm{a}}}{\mathrm{ra}}\frac{\partial {\mathrm{v}}_{ \mathrm{ra}}}{\mathrm{\partial \upvartheta a}}+\frac{{\mathrm{v}}_{\upvarphi \mathrm{a}}}{\mathrm{ra}\ \mathrm{ sin}\upvartheta \mathrm{a}}\frac{\partial {\mathrm{v}}_{\mathrm{ra}}}{\mathrm{\partial \upvarphi a}}-\frac{{\mathrm{ v}}_{\upvartheta \mathrm{a}}^2}{\mathrm{ra}}-\frac{{\mathrm{v}}_{\upvarphi \mathrm{a}}^2}{\mathrm {ra}}+\frac{1}{\uprho}\frac{\mathrm{\partial p}}{\mathrm{\partial ra}}\right]/\left[\frac{1}{{\mathrm {ra}}^2}\frac{\partial }{\mathrm{\partial ra}}\left({\mathrm{ra}}^2\frac{\partial {\mathrm{v}}_{\mathrm {ra}}}{\mathrm{\ra partiel}}\rig ht)+\frac{1}{{\mathrm{ra}}^2\mathrm{sin}\upvartheta \mathrm{a}}\frac{\partial }{\mathrm{\partial \upvartheta a}}\left (\mathrm{sin}\upvartheta \mathrm{a}\frac{\partial {\mathrm{v}}_{\mathrm{ra}}}{\mathrm{\partial \upvartheta a}}\right)+\ frac{1}{{\mathrm{ra}}^2{\sin}^2\upvartheta \mathrm{a}}\frac{\partial^2{\mathrm{v}}_{\mathrm{ra}} }{\partial {\upvarphi \mathrm{a}}^2}-\frac{2{\mathrm{v}}_{\mathrm{ra}}}{{\mathrm{ra}}^2}-\ frac{2}{{\mathrm{ra}}^2\sin \upvartheta \mathrm{a}}\frac{\partial \left({\mathrm{v}}_{\upvartheta \mathrm{a}}\ mathrm{sin}\upvartheta \mathrm{a}\right)}{\mathrm{\partial \upvartheta a}}-\frac{2}{{\mathrm{ra}}^2\mathrm{sin}\upvartheta \ mathrm{a}}\frac{\partial {v}_{\upvarphi \mathrm{a}}}{\partial_{\upvarphi \mathrm{a}}}\right]=\left[\frac{v_{\ upvartheta \mathrm{b}}}{\mathrm{rb}}\frac{\partial {v}_{\mathrm{rb}}}{\mathrm{\partial \upvartheta b}}+\frac{v_{\ upvarphi \mathrm{b}}}{\mathrm{rb}\ \mathrm{sin}\upvartheta \mathrm{b}}\frac{\partial {v}_{\mathrm{rb}}}{\mathrm{\ partiel \ upvarphi b}}-\frac{v_{\upvartheta \mathrm{b}}^2}{\mathrm{rb}}-\frac{v_{\upvarphi \mathrm{b}}^2}{\mathrm{rb }}+\frac{1}{\rho}\frac{\partial p}{\mathrm{\partial rb}}\right]/\left[\frac{1}{{\mathrm{rb}}^2 }\frac{\partial }{\mathrm{\partial rb}}\left({\mathrm{rb}}^2\frac{\partial {v}_{\mathrm{rb}}}{\mathrm{\ partiel rb}}\right)+\frac{1}{{\mathrm{rb}}^2\mathrm{sin}\upvartheta \mathrm{b}}\frac{\partial }{\mathrm{\partial \upvartheta b}}\left(\mathrm{sin}\upvartheta \mathrm{b}\frac{\partial {v}_{\mathrm{rb}}}{\mathrm{\partial \upvartheta b}}\right)+ \frac{1}{{\mathrm{rb}}^2{\sin}^2\upvartheta \mathrm{b}}\frac{\partial^2{v}_{\mathrm{rb}}}{\ partiel {\upvarphi \mathrm{b}}^2}-\frac{2{v}_{\mathrm{rb}}}{{\mathrm{rb}}^2}-\frac{2}{{\ mathrm{rb}}^2\sin \upvartheta \mathrm{b}}\frac{\partial \left({v}_{\upvartheta \mathrm{b}}\mathrm{sin}\upvartheta \mathrm{b} \right)}{\mathrm{\partial \upvartheta b}}-\frac{2}{{\mathrm{rb}}^2\mathrm{sin}\upvartheta \mathrm{b}}\frac{\partial { v}_{\upvarphi \mathrm{b}}}{\partiel _{\upvarphi \mathrm{b}}}\right] $$ (11)L'équation 11 pourrait être représentée comme suit :
$$ \mathrm{d}\upnu =\mathrm{rn}\left[{v}_{\upvartheta \mathrm{n}}\frac{\partial {v}_{\mathrm{rn}}}{\ mathrm{\partial \upvartheta n}}+\frac{v_{\upvarphi \mathrm{n}}}{\ \mathrm{sin}\upvartheta \mathrm{n}}\frac{\partial {v}_{\ mathrm{rn}}}{\mathrm{\partial \upvarphi n}}-{v}_{\upvartheta \mathrm{n}}^2-{v}_{\upvarphi \mathrm{n}}^2+ \frac{\mathrm{rn}}{\rho}\frac{\partial p}{\mathrm{\partial rn}}\right]/\left[\frac{\partial }{\mathrm{\partial rn} }\left({\mathrm{rn}}^2\frac{\partial {v}_{\mathrm{rn}}}{\mathrm{\partial rn}}\right)+\frac{1}{\ mathrm{sin}\upvartheta \mathrm{n}}\frac{\partial }{\mathrm{\partial \upvartheta n}}\left(\mathrm{sin}\upvartheta \mathrm{n}\frac{\partial { v}_{\mathrm{rn}}}{\mathrm{\partial \upvartheta n}}\right)+\frac{1}{\sin^2\upvartheta \mathrm{n}}\frac{\partial^ 2{v}_{\mathrm{rn}}}{\partial {\upvarphi \mathrm{n}}^2}-2{v}_{\mathrm{rn}}-\frac{2}{\sin \upvartheta \mathrm{n}}\frac{\partial \left({v}_{\upvartheta \mathrm{n}}\mathrm{sin}\upvartheta \mathrm{n}\right)}{\mathrm{\ par tial \upvartheta n}}-\frac{2}{\mathrm{sin}\upvartheta \mathrm{n}}\frac{\partial {v}_{\upvarphi \mathrm{n}}}{\partial_{\ upvarphi \mathrm{n}}}\right]=\mathrm{ra}\left[{v}_{\upvartheta \mathrm{a}}\frac{\partial {v}_{\mathrm{ra}}} {\mathrm{\partial \upvartheta a}}+\frac{v_{\upvarphi \mathrm{a}}}{\ \mathrm{sin}\upvartheta \mathrm{a}}\frac{\partial {v}_ {\mathrm{ra}}}{\mathrm{\partial \upvarphi a}}-{v}_{\upvartheta \mathrm{a}}^2-{v}_{\upvarphi \mathrm{a}}^ 2+\frac{\mathrm{ra}}{\rho}\frac{\partial p}{\mathrm{\partial ra}}\right]/\left[\frac{\partial }{\mathrm{\partial ra}}\left({\mathrm{ra}}^2\frac{\partial {v}_{\mathrm{ra}}}{\mathrm{\partial ra}}\right)+\frac{1} {\mathrm{sin}\upvartheta \mathrm{a}}\frac{\partial }{\mathrm{\partial \upvartheta a}}\left(\mathrm{sin}\upvartheta \mathrm{a}\frac{\ partiel {v}_{\mathrm{ra}}}{\mathrm{\partial \upvartheta a}}\right)+\frac{1}{\sin^2\upvartheta \mathrm{a}}\frac{\ partiel^2{v}_{\mathrm{ra}}}{\partial {\upvarphi \mathrm{a}}^2}-2{v}_{\mathrm{ra}}-\frac{2}{ \péché \upvartheta \mathrm{a}}\frac{\partial \left({v}_{\upvartheta \mathrm{a}}\mathrm{sin}\upvartheta \mathrm{a}\right)}{\mathrm{\ partiel \upvartheta a}}-\frac{2}{\mathrm{sin}\upvartheta \mathrm{a}}\frac{\partial {v}_{\upvarphi \mathrm{a}}}{\partial_{\ upvarphi \mathrm{a}}}\right]=\mathrm{rb}\left[{v}_{\upvartheta \mathrm{b}}\frac{\partial {v}_{\mathrm{rb}}} {\mathrm{\partial \upvartheta b}}+\frac{v_{\upvarphi \mathrm{b}}}{\ \mathrm{sin}\upvartheta \mathrm{b}}\frac{\partial {v}_ {\mathrm{rb}}}{\mathrm{\partial \upvarphi b}}-{v}_{\upvartheta \mathrm{b}}^2-{v}_{\upvarphi \mathrm{b}}^ 2+\frac{\mathrm{rb}}{\rho}\frac{\partial p}{\mathrm{\partial rb}}\right]/\left[\frac{\partial }{\mathrm{\partial rb}}\left({\mathrm{rb}}^2\frac{\partial {v}_{\mathrm{rb}}}{\mathrm{\partial rb}}\right)+\frac{1} {\mathrm{sin}\upvartheta \mathrm{b}}\frac{\partial }{\mathrm{\partial \upvartheta b}}\left(\mathrm{sin}\upvartheta \mathrm{b}\frac{\ partiel {v}_{\mathrm{rb}}}{\mathrm{\partial \upvartheta b}}\right)+\frac{1}{\si n^2\upvartheta \mathrm{b}}\frac{\partial^2{v}_{\mathrm{rb}}}{\partial {\upvarphi \mathrm{b}}^2}-2{v} _{\mathrm{rb}}-\frac{2}{\sin \upvartheta \mathrm{b}}\frac{\partial \left({v}_{\upvartheta \mathrm{b}}\mathrm{sin }\upvartheta \mathrm{b}\right)}{\mathrm{\partial \upvartheta b}}-\frac{2}{\mathrm{sin}\upvartheta \mathrm{b}}\frac{\partial {v }_{\upvarphi \mathrm{b}}}{\partial_{\upvarphi \mathrm{b}}}\right] $$ (12)Il existe une relation directe entre les rayons des nanoparticules et la viscosité lymphatique due au cancer. Si la lymphe devient trop statique et visqueuse, elle est incapable de remplir correctement sa fonction, qui est de faire circuler et de nettoyer les toxines et d'aider à lutter contre le cancer. Si la taille des nanoparticules est plus petite, les cellules cancéreuses lymphatiques sont faciles à tuer. Afin de décrire le transport de la quantité totale des nanoparticules composées, nous utilisons l'équation de continuité et supposons les trois nanoparticules de solides A, B et N comme suit :
$$ \frac{1}{{\mathrm{ra}}^2}\frac{\partial }{\mathrm{\partial ra}}\left({\mathrm{ra}}^2{v}_{ \mathrm{ra}}\right)+\frac{1}{\mathrm{ra}\ \mathrm{sin}\upvartheta \mathrm{a}}\frac{\partial }{\mathrm{\partial \upvartheta a }}\left(\sin {\upvartheta \mathrm{v}}_{\upvartheta \mathrm{a}}\right)+\frac{1}{\mathrm{ra}\ \mathrm{sin}\upvartheta \ mathrm{a}}\frac{\partial {v}_{\upvarphi \mathrm{a}}}{\mathrm{\partial \upvarphi a}}+\frac{1}{{\mathrm{rb}}^ 2}\frac{\partial }{\mathrm{\partial rb}}\left({\mathrm{rb}}^2{v}_{\mathrm{rb}}\right)+\frac{1}{ \mathrm{rb}\ \mathrm{sin}\upvartheta \mathrm{b}}\frac{\partial }{\mathrm{\partial \upvartheta b}}\left(\sin {\upvartheta v}_{\upvartheta \mathrm{b}}\right)+\frac{1}{\mathrm{rb}\ \mathrm{sin}\upvartheta \mathrm{b}}\frac{\partial {v}_{\upvarphi \mathrm{ b}}}{\mathrm{\partial \upvarphi b}}+\frac{1}{{\mathrm{rn}}^2}\frac{\partial }{\mathrm{\partial rn}}\left( {\mathrm{rn}}^2{v}_{\mathrm{rn}}\right)+\frac{1}{\mathrm{rn}\ \mathrm{sin}\upvartheta \mathrm{n}}\ frac{\partie al }{\mathrm{\partial \upvartheta n}}\left(\sin {\upvartheta v}_{\upvartheta \mathrm{n}}\right)+\frac{1}{\mathrm{rn}\ \ mathrm{sin}\upvartheta \mathrm{n}}\frac{\partial {v}_{\upvarphi \mathrm{n}}}{\mathrm{\partial \upvarphi n}}=0 $$ (13)La viscosité dynamique du fluide peut être déterminée à partir de l'équation suivante [23] :
$$ \mathrm{Vs}=\frac{2}{9}\frac{r^2g\ \left(\uprho \mathrm{p}-\uprho \mathrm{f}\right)}{\mathrm{dv }} $$ (14)where Vs is the particles’ settling velocity (m/s), r is the Stokes radius of the particle (m), g is the gravitational acceleration (m/s 2 ), ρp is the density of the particles (kg/m 3 ), ρf is the density of the fluid (kg/m 3 ), and dv is the (dynamic) fluid viscosity (Pa·s). The lymph fluid is slightly heavier than water (lymph density = 1019 kg/m 3 , water density = 998.28 kg/m 3 at 20 °C). As a reference value, we consider the dynamic viscosity of the water to be 1.002 × 10 –3 kg m –1 s –1 ).
Dynamic viscosity is the measurement of the fluid’s internal resistance to flow, while kinematic viscosity refers to the ratio of dynamic viscosity to density. The effect of all the nanoparticles on the fluid viscosity is represented as follows:
$$ \mathrm{dv}=\frac{2\mathrm{g}}{9}\left[\frac{{\mathrm{ra}}^2\left(\uprho \mathrm{a}-\uprho \mathrm{f}\right)}{\mathrm{vsa}}+\frac{{\mathrm{rb}}^2\left(\uprho \mathrm{b}-\uprho \mathrm{f}\right)}{\mathrm{vsb}}+\frac{{\mathrm{rn}}^2\left(\uprho \mathrm{n}-\uprho \mathrm{f}\right)}{\mathrm{vn}}\right] $$ (15)By comparing Eq. 12 and Eq. 15, the following equation could be emerged:
$$ \left|\frac{2\mathrm{g}}{9}\left[\frac{{\mathrm{ra}}^2\left(\uprho \mathrm{a}-\uprho \mathrm{f}\right)}{\mathrm{vsa}}+\frac{{\mathrm{rb}}^2\left(\uprho \mathrm{b}-\uprho \mathrm{f}\right)}{\mathrm{vsb}}+\frac{{\mathrm{rn}}^2\left(\uprho \mathrm{n}-\uprho \mathrm{f}\right)}{\mathrm{vn}}\right]\right|=\mathrm{rn}\left[{v}_{\upvartheta \mathrm{n}}\frac{\partial {v}_{\mathrm{rn}}}{\mathrm{\partial \upvartheta n}}+\frac{v_{\upvarphi \mathrm{n}}}{\ \mathrm{sin}\upvartheta \mathrm{n}}\frac{\partial {v}_{\mathrm{rn}}}{\mathrm{\partial \upvarphi n}}-{v}_{\upvartheta \mathrm{n}}^2-{v}_{\upvarphi \mathrm{n}}^2+\frac{\mathrm{rn}}{\uprho \mathrm{f}}\frac{\mathrm{\partial p}}{\mathrm{\partial rn}}\right]/\left[\frac{\partial }{\mathrm{\partial rn}}\left({\mathrm{rn}}^2\frac{\partial {v}_{\mathrm{rn}}}{\mathrm{\partial rn}}\right)+\frac{1}{\mathrm{sin}\upvartheta \mathrm{n}}\frac{\partial }{\mathrm{\partial \upvartheta n}}\left(\mathrm{sin}\upvartheta \mathrm{n}\frac{\partial {v}_{\mathrm{rn}}}{\mathrm{\partial \upvartheta n}}\right)+\frac{1}{\sin^2\upvartheta \mathrm{n}}\frac{\partial^2{v}_{\mathrm{rn}}}{\partial {\upvarphi \mathrm{n}}^2}-2{v}_{\mathrm{rn}}-\frac{2}{\sin \upvartheta \mathrm{n}}\frac{\partial \left({v}_{\upvartheta \mathrm{n}}\mathrm{sin}\upvartheta \mathrm{n}\right)}{\mathrm{\partial \upvartheta n}}-\frac{2}{\mathrm{sin}\upvartheta \mathrm{n}}\frac{\partial {v}_{\upvarphi \mathrm{n}}}{\partial_{\upvarphi \mathrm{n}}}\right]=\mathrm{ra}\left[{v}_{\upvartheta \mathrm{a}}\frac{\partial {v}_{\mathrm{ra}}}{\mathrm{\partial \upvartheta a}}+\frac{v_{\upvarphi \mathrm{a}}}{\ \mathrm{sin}\upvartheta \mathrm{a}}\frac{\partial {v}_{\mathrm{ra}}}{\mathrm{\partial \upvarphi a}}-{v}_{\upvartheta \mathrm{a}}^2-{v}_{\upvarphi \mathrm{a}}^2+\frac{\mathrm{ra}}{\uprho \mathrm{f}}\frac{\partial p}{\mathrm{\partial ra}}\right]/\left[\frac{\partial }{\mathrm{\partial ra}}\left({\mathrm{ra}}^2\frac{\partial {v}_{\mathrm{ra}}}{\mathrm{\partial ra}}\right)+\frac{1}{\mathrm{sin}\upvartheta \mathrm{a}}\frac{\partial }{\mathrm{\partial \upvartheta a}}\left(\mathrm{sin}\upvartheta \mathrm{a}\frac{\partial {v}_{\mathrm{ra}}}{\mathrm{\partial \upvartheta a}}\right)+\frac{1}{\sin^2\upvartheta \mathrm{a}}\frac{\partial^2{v}_{\mathrm{ra}}}{\partial {\upvarphi \mathrm{a}}^2}-2{v}_{\mathrm{ra}}-\frac{2}{\sin \upvartheta \mathrm{a}}\frac{\partial \left({v}_{\upvartheta \mathrm{a}}\mathrm{sin}\upvartheta \mathrm{a}\right)}{\mathrm{\partial \upvartheta a}}-\frac{2}{\mathrm{sin}\upvartheta \mathrm{a}}\frac{\partial {v}_{\upvarphi \mathrm{a}}}{\partial_{\upvarphi \mathrm{a}}}\right]=\mathrm{rb}\left[{v}_{\upvartheta \mathrm{b}}\frac{\partial {v}_{\mathrm{rb}}}{\mathrm{\partial \upvartheta b}}+\frac{v_{\upvarphi \mathrm{b}}}{\ \mathrm{sin}\upvartheta \mathrm{b}}\frac{\partial {v}_{\mathrm{rb}}}{\mathrm{\partial \upvarphi b}}-{v}_{\upvartheta \mathrm{b}}^2-{v}_{\upvarphi \mathrm{b}}^2+\frac{\mathrm{rb}}{\uprho \mathrm{f}}\frac{\partial p}{\mathrm{\partial rb}}\right]/\left[\frac{\partial }{\mathrm{\partial rb}}\left({\mathrm{rb}}^2\frac{\partial {v}_{\mathrm{rb}}}{\mathrm{\partial rb}}\right)+\frac{1}{\mathrm{sin}\upvartheta \mathrm{b}}\frac{\partial }{\mathrm{\partial \upvartheta b}}\left(\mathrm{sin}\upvartheta \mathrm{b}\frac{\partial {v}_{\mathrm{rb}}}{\mathrm{\partial \upvartheta b}}\right)+\frac{1}{\sin^2\upvartheta \mathrm{b}}\frac{\partial^2{v}_{\mathrm{rb}}}{\partial {\upvarphi \mathrm{b}}^2}-2{v}_{\mathrm{rb}}-\frac{2}{\sin \upvartheta \mathrm{b}}\frac{\partial \left({v}_{\upvartheta \mathrm{b}}\mathrm{sin}\upvartheta \mathrm{b}\right)}{\mathrm{\partial \upvartheta b}}-\frac{2}{\mathrm{sin}\upvartheta \mathrm{b}}\frac{\partial {v}_{\upvarphi \mathrm{b}}}{\partial_{\upvarphi \mathrm{b}}}\right] $$ (16)Equation 16 depicts the relationship between the density of the lymph fluid, the density of the nanoparticles of the compound drug system, and the radius of the nanoparticles. There is a positive correlation between the density of the lymph fluid and the density of the nanoparticles. The smaller the density and radius of nanoparticles, the lesser the density of the fluid will be. As established earlier, the decrease in the density of the lymph fluid leads to its inability to reproduce and reduce the ferocity of the disease. It can, therefore, be concluded that the tumor can be cured by minimizing the size of nanoparticles. These particles can reach in the range of up to 0.1 nm (i.e., Angstrom or picometer range). The particles of this size can act as the nucleus of drug delivery in this drug system. Equation 16 shows that radii of the nanoparticles in the proposed drug system are related to the effectiveness of the delivery system. Much lower sized nanoparticles can reduce the density of lymph fluid and the spread of the disease.
Nanoparticles with Nanoantennas
This study used the nanoparticle described in an earlier report [22] as an emissary with a nano-microcontroller. In the system, the proposed transmission distance is very small and compatible with the composition of nanoparticles. Thus, the middle gap can be neglected in mid-distance and is symbolized by C d . Further, R un et X un are the real part and the imaginary part of the anaerobic impedance. After neglecting the load of the intercellular space between the nanoparticles and the nano-microcontroller, R un et X un can be calculated as follows [22]:
$$ {R}_a=\frac{r_{a0}}{1+{C}_d{w}_a\left(2{x}_{a0}+{C}_d\left({r_{a0}}^2+{x_{a0}}^2\right){w}_a\right)} $$ (17) $$ {X}_a=\frac{x_0-{C}_d\left({r_{a0}}^2+{x_{a0}}^2\right){w}_a}{1+{C}_d{w}_a\left(2{x}_{a0}+{C}_d\left({r_{a0}}^2+{x_{a0}}^2\right){w}_a\right)} $$ (18)Thus, the load resistance value of nanotubes can be predicted as in the following equation:
$$ {r}_l=\frac{g^2R}{g^2-2 gSX{\varepsilon}_L\omega +{S}^2\left({R}^2+{X}^2\right){\varepsilon_L}^2{\omega}^2} $$ (19)où ε L is the permittivity of the loading material, g is the size of the gap, and S is the effective cross-section area of the gap. In order to simplify the equation, the value of g 2 can be neglected as it is too low and the final equation can be rewritten as follows:
$$ {r}_l=\frac{g^2R}{-2 gSX{\varepsilon}_L\omega +{S}^2\left({R}^2+{X}^2\right){\varepsilon_L}^2{\omega}^2} $$ (20)Then,
$$ {r}_l=\frac{g^2R}{S{\varepsilon}_L\omega \left(-2 gX+S\left({R}^2+{X}^2\right){\varepsilon}_L\omega \right)} $$ (21)The optical nano-photo concept can be used as an effective tool for interpreting and predicting these effects to design and improve nanoscale parameters and increase the nano-sensitivity to serve better as a single molecular sensor. Nanoantenna may provide optimal performance in terms of sensitivity, efficiency, and bandwidth in the process. The next section presents the concept of searching the cancerous lymph nodes using compressive binary search algorithm.
Searching for the Target Lymphatic Nodes Using Compressive Binary Search
In order for nanoparticles to reach the cancerous cells in a fast and efficient manner, we applied compressive binary search by the nano-microcontroller. The guided nanoparticles follow a specific path to quickly reach the target. This movement is based on the information obtained from the “exploratory nanoparticles.” Assume that the target lymph node, Tf, has exactly one nonzero entry, where the location of the lymph node is unknown. The algorithm divides mt measurements into a total of St stages, where St refers to the stages of the lymph nodes. The measurements are more than one for all the stages of the lymph nodes, which is necessary for the algorithm to be executed until completion. Based on this measurement, the algorithm decides between going left or right, until the nanoparticles reach the target, the cancerous lymph node.
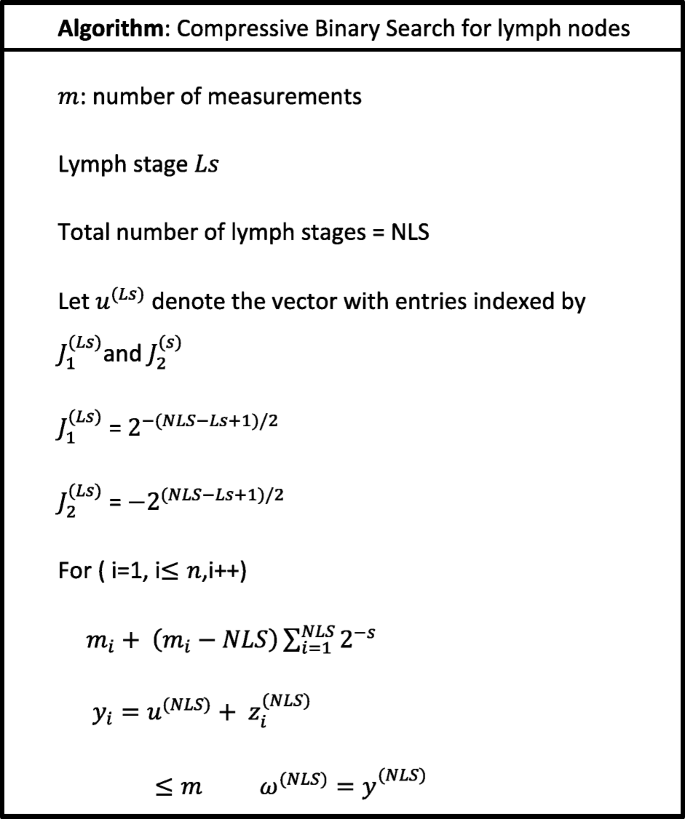
Résultats et discussion
In order to analyze the proposed design, the nanoparticles were applied to the following five types of materials:silicone, lithium, lung, helium, and hydrogen. The materials were chosen because of their low density. The lung nanoparticles were samples from nano-sized lung nodules. They appear encircling with white shadows in a chest X-ray or computerized tomography scan taken from the lung of the person and required to be undamaged. The proposed idea is based on the analytical model, which indicates that the smaller the density of nanoparticles, the smaller the dynamic viscosity will be. This will result in a decrease in fluid viscosity. It is shown that the types of materials and the density of each particle will affect settling velocity of nanoparticles at entry into the lymphatic fluid and the density of the lymphatic fluid. We considered the following parameters:acceleration of gravity (g ) = 9.80665, particle diameter (d ) = 10 A, initial density of lymph fluid (ρf) = 998.28, and dynamic viscosity = 0.0010 kg m –1 s –1 [24]. These parameters were selected by the assumption that the viscosity of the lymphatic fluid is very similar to the viscosity of the water and the very small difference does not affect the results of the model. Figure 4 illustrates the density of nanoparticles for five selected materials for application in the proposed analytical model. Figure 5 shows the settling velocity for each particle. Figure 6 shows the effect of the settling velocity of nanoparticles on altering the lymphocyte density of cancer cells. The results shown in Fig. 6 show that the settling velocity of the particles carries a negative value. This indicates that the nanoparticle after entering in the lymphatic fluid rapidly moves in the opposite direction toward their entry into the lymphatic fluid. In general, any object that moves in the negative direction has a negative velocity. This movement of the particle leads to reduced viscosity of the lymphatic fluid.
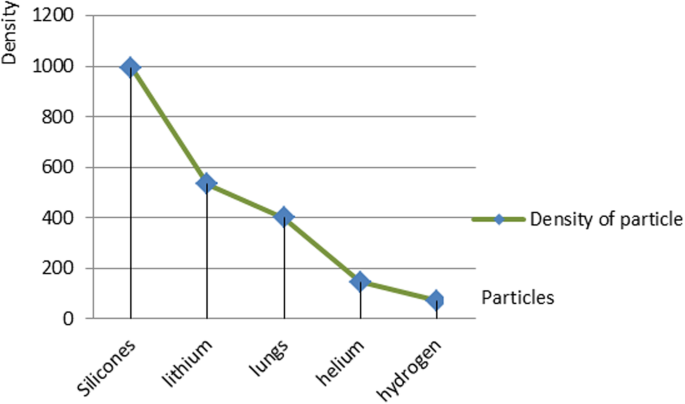
Density of nanoparticles for the five selected materials
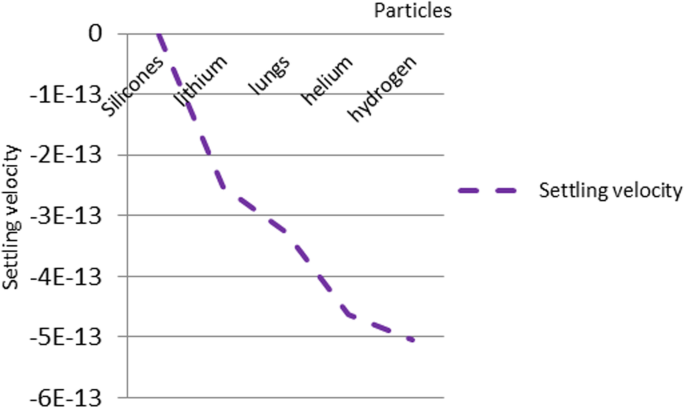
The settling velocity data for each particle
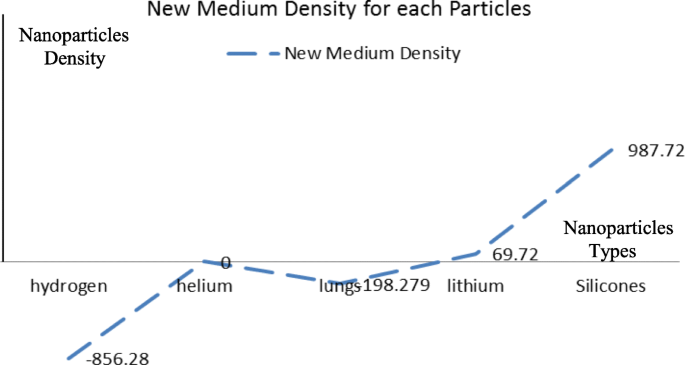
The effect of the settling velocity of nanoparticles on changing the lymphocyte density of cancer cells
Silicone nanoparticles showed the settling velocity of approximately − 2.87 × 10–15 m/s. This resulted in a decrease in viscosity of the lymphatic fluid to 987.72 kg/m 3 for the initial density 998.28 kg/m 3 . The density is continuously reduced to a point where hydrogen produces extremely spectacular results, i.e., the complete collapse of lymphatic fluid resistance. The density of the lymphatic fluid − 856.28 kg/m 3 with the negative sign indicated that there was no resistance from the lymphatic fluid to the flow of the nanoparticles, resulting in the complete collapse of the liquid fluid. Both the hydrogen and helium particles have a significant impact on the liquid viscosity due to the low density of the particles. Hence, it is important to use a drug system consisting of a group of nanoparticles for low-density materials. Figure 7 shows the relationship between the diameters of lung nanoparticles and the number of nanoparticles in one group. The figure shows that the higher the diameter of nanoparticles, the fewer their number in a group. This is clearly shown at the highest value of the nanoparticle diameter of 1000 nm, where the number of molecules in a group is 20 molecules. Figure 8 shows the relationship between the diameters of lithium nanoparticles and the number of nanoparticles in one group. This figure demonstrates the inverse relationship between the radius of nanoparticles and the number of molecules in a group where lithium particle diameters are significantly lower than the lung nanoparticles, where the number of nanoparticles in Fig. 7 is relatively low compared to the lithium particles as shown in Fig. 8. And the multicolor balls in both figures refer to different ranges of nanoparticle radii for each group, where each group contains a number of nanoparticles with different sizes. The best results can be obtained when hydrogen and helium particles are increased from other substances. A mixture of different materials should be used so that the properties of these substances can be used in the treatment process as well as to reduce viscosity. Figure 9 illustrates the different sets of materials proposed to have the mean highest density of both hydrogen and helium materials. Figure 10 shows the average mass of a nanoparticle in a group. It can be seen that the mass of both hydrogen and helium is the highest compared to the mass of particles of other substances. Figure 11 illustrates the relationship between the diameters of the nanoparticles and the width of its group or class. It is important to note that these results will open up a new area to reduce the resistance of the lymphatic fluid in tumors. This can be achieved using hydrogen nanoparticles of a size in the range of Angstrom. In addition to hydrogen nanoparticles, there may also exist a number of other substances in the same size. Figure 12 illustrates the standard deviation of a number of coefficients for both lung and lithium nanoparticles. These coefficients are limited to fractions of nanoparticles in a single group as well as their number in addition to the diameters of these nanoparticles. It is clear that the group fractions have the less value of the standard deviation. Hence, most of the fractions in the computational processes are around the mean of these values. Figure 13 shows the standard deviation of the mass for particles of silicones, lithium, lungs, helium, and hydrogen in one group. It is clear that the particles of the lung have the largest standard deviation and the lithium has the minimum value.
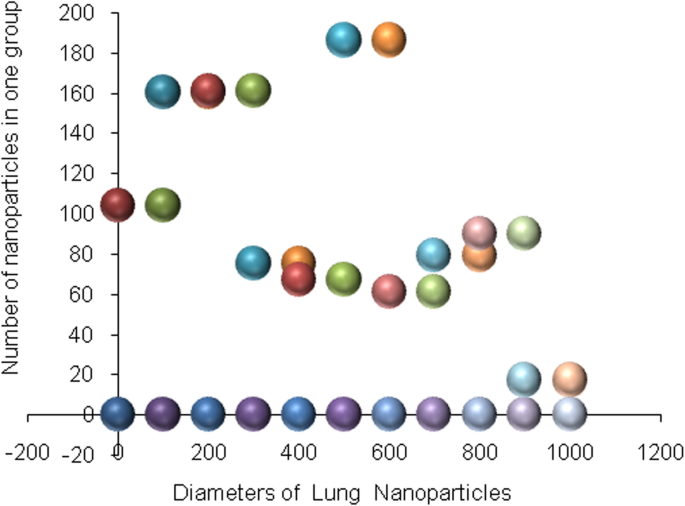
Group of nanoparticles in the lung cells and their number in one of the proposed groups
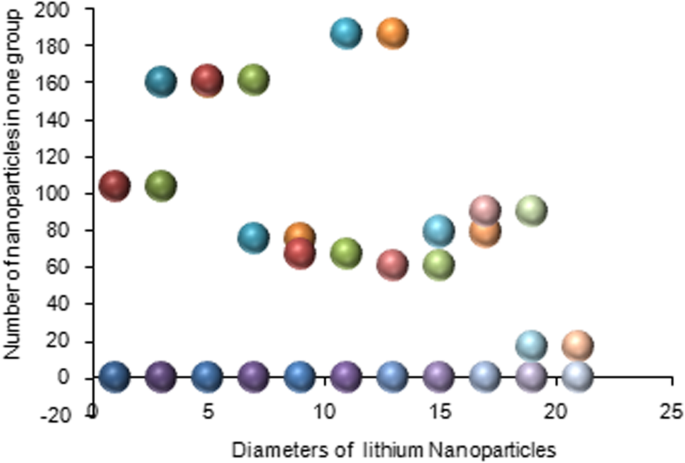
Group of nanoparticles in the lithium cells and their number in one of the proposed groups
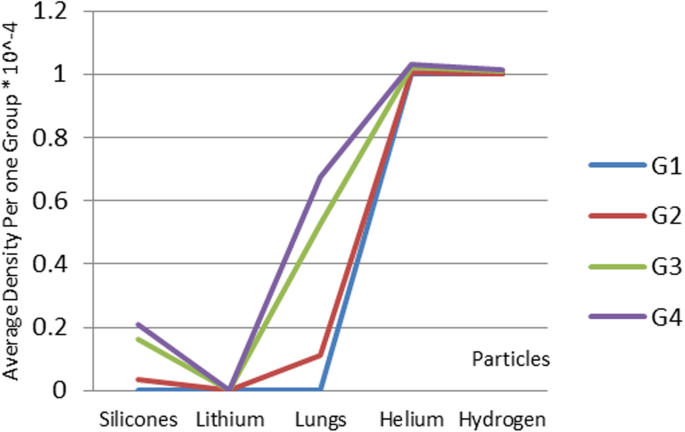
Different sets of materials proposed to have the mean highest density of both hydrogen and helium materials
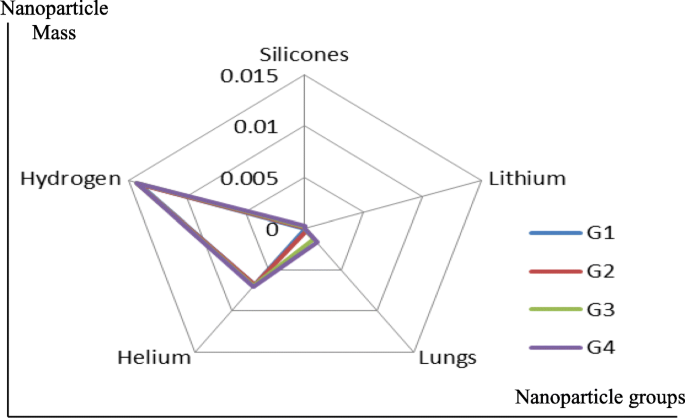
Average mass of a nanoparticle in a group
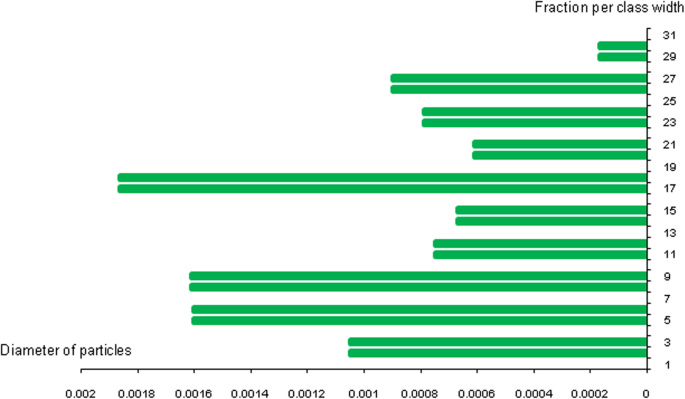
Diameters of the nanoparticles related to the group width
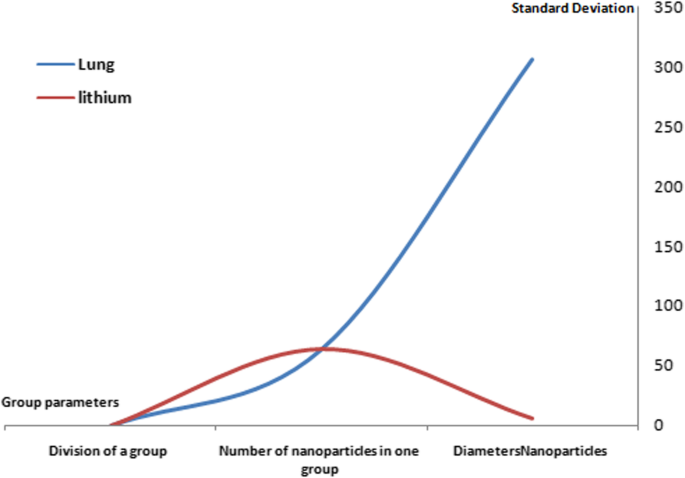
The standard deviation of lung and lithium nanoparticles coefficients
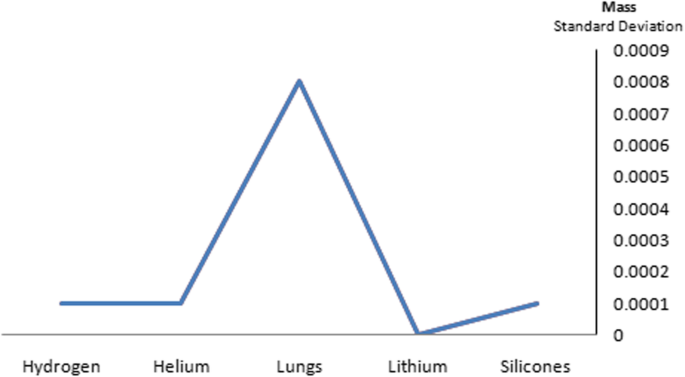
The standard deviation of the mass for particles of silicones, lithium, lungs, helium, and hydrogen in one group
Méthodes
The aim of this study was to establish a nano-drug delivery system capable of delivering the drugs effectively to the cancer cells. The following methodology was used to deliver nanoparticles:
- i)
Low-density nanoparticles
This study proposed the theoretical approach of nanoparticles as a low-density drug. This depends on the density and the settling velocity of the nanoparticles, as these nanoparticles can overcome the resistance of the lymphatic fluid.
- ii)
Preparation of anaerobic nanoparticles
This study uses the idea of nanoparticles possessing an antenna through which a connection can be made between nanoparticles and nano-controller. The transmission distance was assumed to be too small to match the composition of nanoparticles and also to fit the actual distance between them.
- iii)
Nano-controller design
Its function is to deliver the nanoparticle drug to cancer cells. Its role is to send signals to the nanoparticles and coordinate their actions and direct them to the lymphatic fluid of tumors.
- iv)
Searching for the target lymphatic nodes
The lymphatic nodes are searched using compressive binary search algorithm. This algorithm is characterized by high-speed search, which makes nanoparticles more accessible to infected cells than the conventional methods. The primary supervisor behind the performance of the nanoparticles is the nano-controller. It directs nanoparticles to the infected cells by following this algorithm to ensure that an appropriate number of molecules are in proportional density to the lymphatic fluid.
Conclusion
There have been various studies managing the treatment of malignant growth utilizing nanoparticles. The lymphatic liquid in tumors plays a substantial role in the obstruction of medication to the cancer cells. We developed an intelligent drug delivery system containing a consortium of nanoparticles. The proposed design demonstrates that small nanoparticles result in low density of the fluid. The results indicated that hydrogen particles are most efficient in reducing resistance toward lymphatic liquid owing to their smaller size. Furthermore, the design involves an anaerobic nano-controller that can determine the state and area of the particles. This technique conveys the medication to the infected cell more effectively.
Disponibilité des données et des matériaux
The datasets supporting the results of this article are included within the article.
Abréviations
- LN:
-
Lipid nanoparticles
- NLC:
-
Nanostructured lipid carriers
- PN:
-
Polymeric nanoparticles
- SLNs:
-
Solid lipid nanoparticles
Nanomatériaux
- Conception à sécurité intégrée
- Nanofibres et filaments pour une administration améliorée des médicaments
- Nanoparticules FePO4 biocompatibles :administration de médicaments, stabilisation de l'ARN et activité fonctionnelle
- Cadres métallo-organiques sensibles à l'environnement comme système d'administration de médicaments pour la thérapie tumorale
- Distribution de médicaments à base de cellules pour les applications contre le cancer
- Zebrafish :Un système modèle en temps réel prometteur pour l'administration de médicaments neurospécifiques par la nanotechnologie
- 131I-Tracé PLGA-Lipid Nanoparticles as Drug Delivery Carriers for the Targeted Chemotherapeutic Treatment of Melanoma
- Étude d'un nouveau système micellaire ressemblant à un ver amélioré par des nanoparticules
- Nanotechnologie :du système d'imagerie in Vivo à l'administration contrôlée de médicaments