Quels sont les types d'imprimantes 3D et que peuvent-elles faire ?
L'impression 3D est une méthode de fabrication optimale pour produire des prototypes et même certaines pièces d'utilisation finale. C'est souvent l'option la plus rentable et offre une valeur considérable, en fonction de la technologie spécifique que vous choisissez.
Il existe de nombreuses catégories d'imprimantes 3D et parmi ces nombreux types d'imprimantes commerciales et industrielles. Cet article couvre les principaux types d'imprimantes 3D et ce qu'elles sont capables de produire.
Comprendre le paysage complexe de l'impression 3D vous aidera au moment de fabriquer les pièces que vous avez conçues et vous fera économiser beaucoup de temps et d'argent. Alors, commençons.
Quels sont les différents types d'imprimantes 3D ?
En 2015, l'ISO/ASTM 52900 a été créée pour normaliser la classification des imprimantes 3D et de la technologie d'impression 3D. Au total, sept catégories de processus ont été établies.
-
Polymérisation en cuve : photopolymère liquide (résine) photopolymérisable
-
Extrusion de matériau : thermoplastique fondu (filament) déposé à travers une buse chauffée
-
Fusion sur lit de poudre (PBF) : particules de poudre fusionnées par une source de haute énergie
-
Jet de matière : gouttelettes d'agent de fusion photosensible liquide déposées sur un lit de poudre et photopolymérisées
-
Jet de liant : gouttelettes de liant liquide déposées sur un lit de matériaux granulés, qui sont ensuite frittés ensemble
-
Dépôt direct d'énergie : métal en fusion déposé et fusionné simultanément
-
Laminage de feuilles : feuilles individuelles de matériau découpées à la forme et laminées ensemble.
Chaque parapluie technologique comprend de nombreux types différents de technologies et d'imprimantes. Les processus que nous proposons dans les hubs sont la stéréolithographie (SLA), la modélisation par dépôt de fil fondu (FDM), le frittage sélectif par laser (SLS) et la fusion multi-jets HP (MJF).
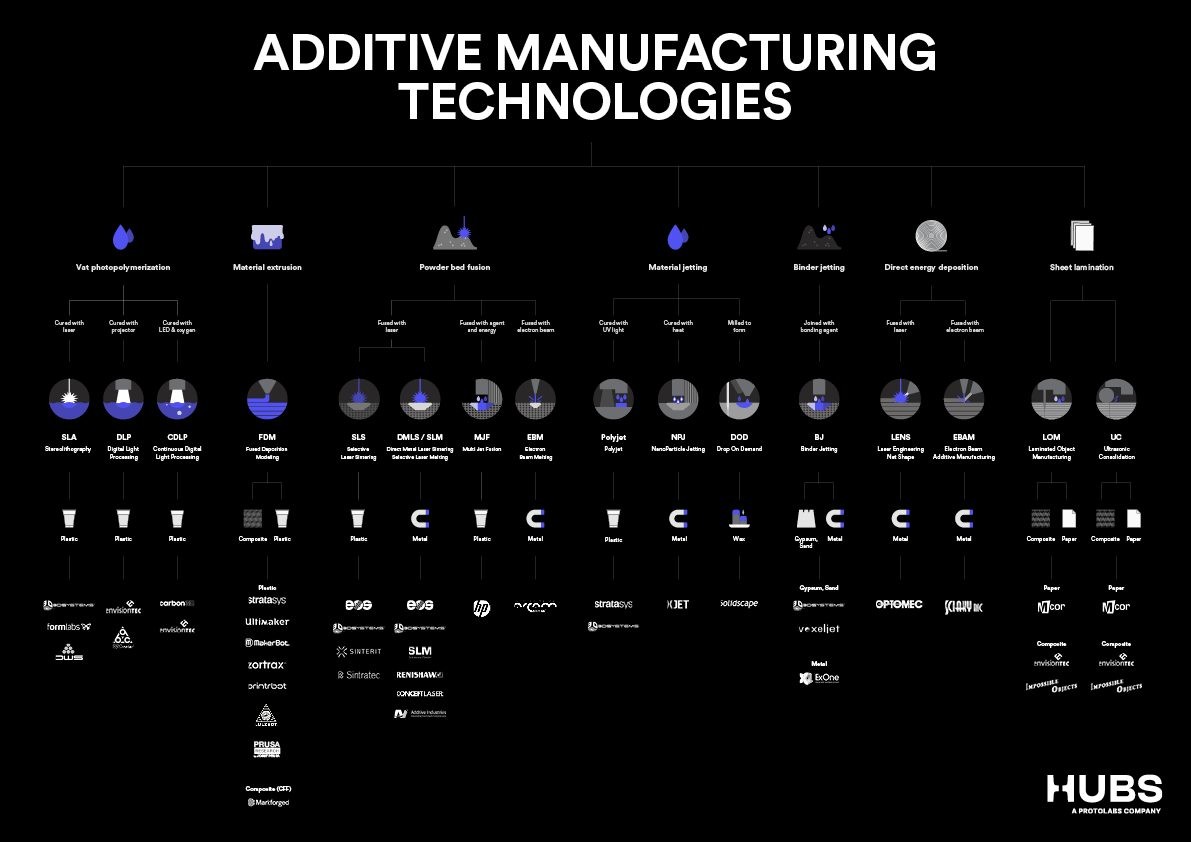
Qu'est-ce que la photopolymérisation en cuve et comment ça marche ?
La photopolymérisation en cuve produit des pièces en durcissant sélectivement des résines photopolymères liquides avec une source de lumière UV. Une plate-forme de construction est immergée dans un réservoir rempli de résine. La lumière est sélectivement dirigée sur la surface de la résine avec des miroirs.
Une fois qu'une couche est durcie, la plate-forme est élevée ou abaissée par petits incréments pour permettre au nouveau liquide de s'écouler. La couche suivante est ensuite polymérisée et jouxte celle précédemment polymérisée. Une fois la couche finale durcie, l'impression est retirée de la résine. À ce stade, il est complètement formé mais peut être renforcé avec un durcissement supplémentaire dans un four UV.
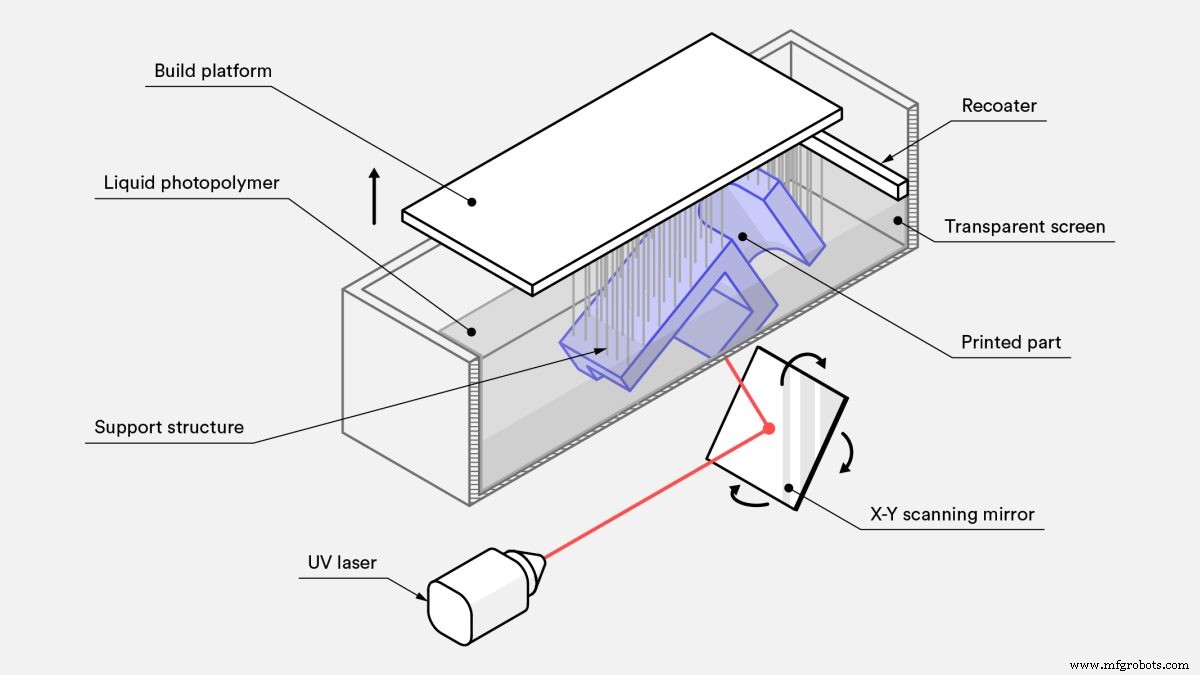
Types d'imprimantes 3D à photopolymérisation en cuve
La photopolymérisation en cuve a quelques technologies d'impression distinctes. Les trois plus courants sont le SLA, le traitement direct de la lumière (DLP ) et production continue d'interface liquide (CLIP). Ils sont très similaires en ce qui concerne la manière dont une source lumineuse dirige la lumière sur la résine. Dans l'ensemble, le SLA est le plus courant et le plus largement utilisé parmi les imprimantes de la famille de la photopolymérisation en cuve.
Détaillons les différents types d'imprimantes 3D à photopolymérisation :
-
SLA utilise un laser à point unique pour tracer une fine ligne le long de la surface de la résine, remplissant la forme de la couche transversale à durcir. Il est très précis mais peut prendre du temps.
-
DLP utilise un projecteur de lumière numérique pour flasher une seule image d'une couche entière en une seule fois. Cela le rend plus rapide que SLA. Cependant, comme le projecteur est un écran numérique, l'image de chaque couche est composée de pixels carrés, ce qui entraîne une résolution légèrement inférieure.
-
CLIP est identique à DLP, sauf que la plate-forme de construction se déplace dans un mouvement continu. Cela permet des temps de construction encore plus rapides et des contours plus lisses le long de l'axe z.
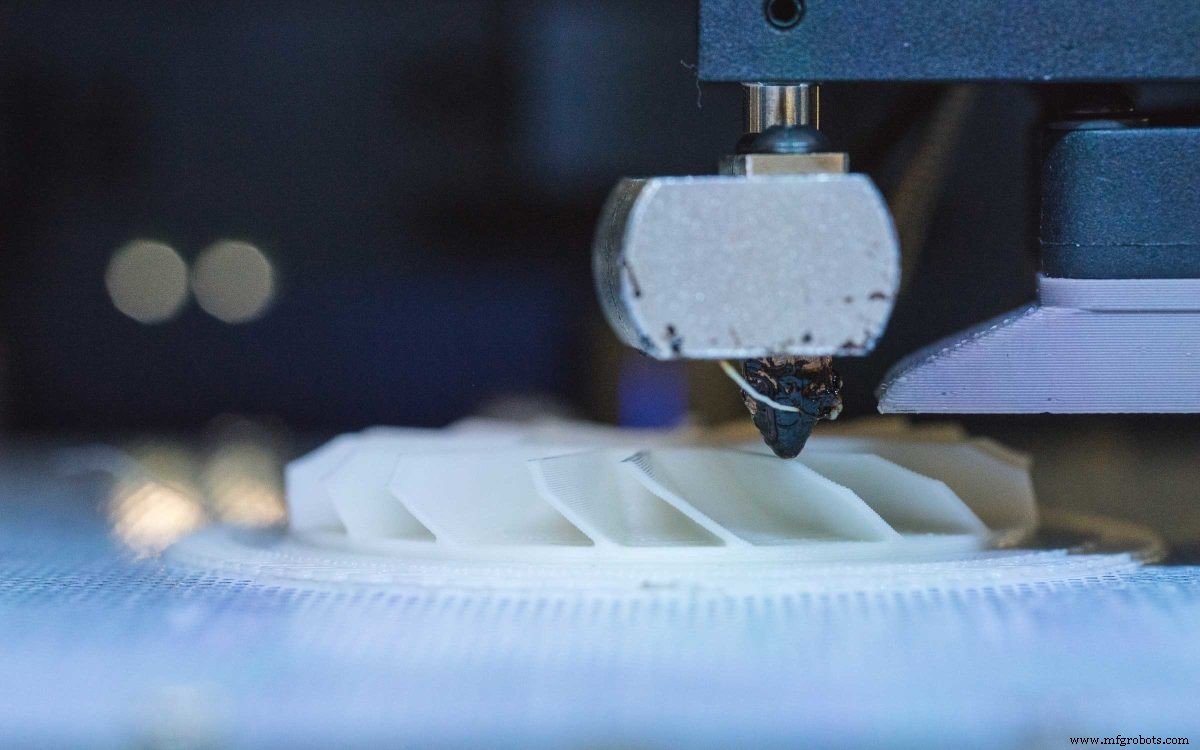
Matériaux pour la photopolymérisation en cuve
Toutes les imprimantes à photopolymérisation en cuve utilisent des résines photopolymères, dont la plupart sont propriétaires. Il existe de nombreux types différents, y compris des résines standard pour le prototypage à usage général. Les autres types courants incluent l'ABS résistant -like résines, résines souples caoutchouteuses, résines coulables transparentes sans teneur en cendres après combustion, résines chargées céramiques pour des impressions très rigides et résines biocompatibles pour dispositifs médicaux.
Certaines résines, y compris les résines transparentes, nécessitent un post-traitement supplémentaire pour obtenir les meilleurs résultats visuels ou, dans le cas des résines transparentes, la clarté optique. Si vous optez pour le SLA pour vos pièces personnalisées, consultez notre comparaison des différents matériaux d'impression 3D SLA .
Propriétés géométriques de la photopolymérisation en cuve
Les volumes de fabrication varient considérablement entre les imprimantes SLA de bureau et les imprimantes SLA industrielles, mais sont généralement inférieurs à ceux des imprimantes FDM ou PBF. Ils impriment un maximum d'environ 300 mm dans n'importe quelle dimension. Les machines de photopolymérisation en cuve peuvent imprimer des pièces très complexes, mais pas aussi complexes que celles réalisées par PBF en raison du besoin de structures de support.
Ce qui lui manque en termes de volume et de complexité de construction, la photopolymérisation en cuve le compense dans la résolution d'impression, car ces machines peuvent imprimer des détails incroyablement fins.
Propriétés mécaniques de la photopolymérisation en cuve
Les imprimantes à photopolymérisation en cuve produisent des pièces avec des tolérances très strictes et peuvent permettre une répétabilité constante. Si les pièces sont entièrement polymérisées après l'impression, elles peuvent également être entièrement isotropes. Mais là où cette technologie brille vraiment, c'est dans la finition de surface. La photopolymérisation en cuve peut imprimer des contours extrêmement lisses, comparables à ce que le moulage par injection peut atteindre.
Un autre avantage majeur par rapport aux autres technologies est que les pièces entièrement durcies peuvent être rendues étanches à l'eau et à l'air. Cependant, le processus de durcissement est irréversible, de sorte que les pièces chauffées brûlent au lieu de fondre.
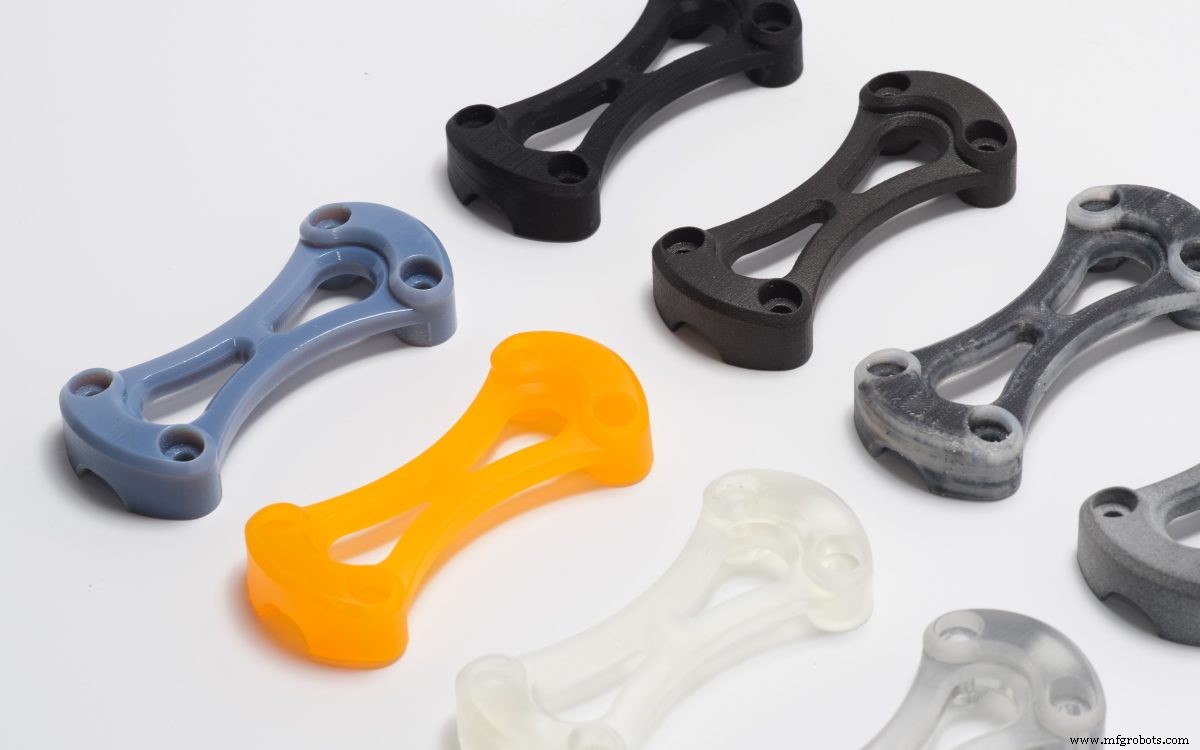
Les imprimantes à photopolymérisation de cuve sont-elles rapides et économiques ?
La polymérisation en cuve est le type d'impression 3D le plus ancien, les premières imprimantes SLA remontant aux années 1970. La concurrence entre les fabricants d'imprimantes continue de faire baisser les prix, mais elle reste chère, coûtant à peu près le même prix que la fusion sur lit de poudre plastique (PBF). Ces machines sont moins chères que celles pour SLS, mais le matériel est plus cher.
De nombreux facteurs affectent les temps d'impression, mais la polymérisation en cuve est généralement considérée comme l'une des technologies les plus rapides.
Applications commerciales :que pouvez-vous produire avec la photopolymérisation en cuve ?
La large gamme de matériaux disponibles offre aux procédés de photopolymérisation en cuve une gamme d'applications tout aussi étendue, du prototypage aux pièces d'utilisation finale.
La haute résolution et les tolérances serrées rendent la technologie idéale pour les bijoux , moulage par injection en petite série et de nombreuses applications dentaires et médicales . La possibilité d'imprimer des pièces étanches rend la photopolymérisation en cuve populaire dans les secteurs de l'automobile, de l'aérospatiale et de la santé. De plus, les finitions de surface lisses le rendent optimal pour le prototypage de pièces moulées par injection.
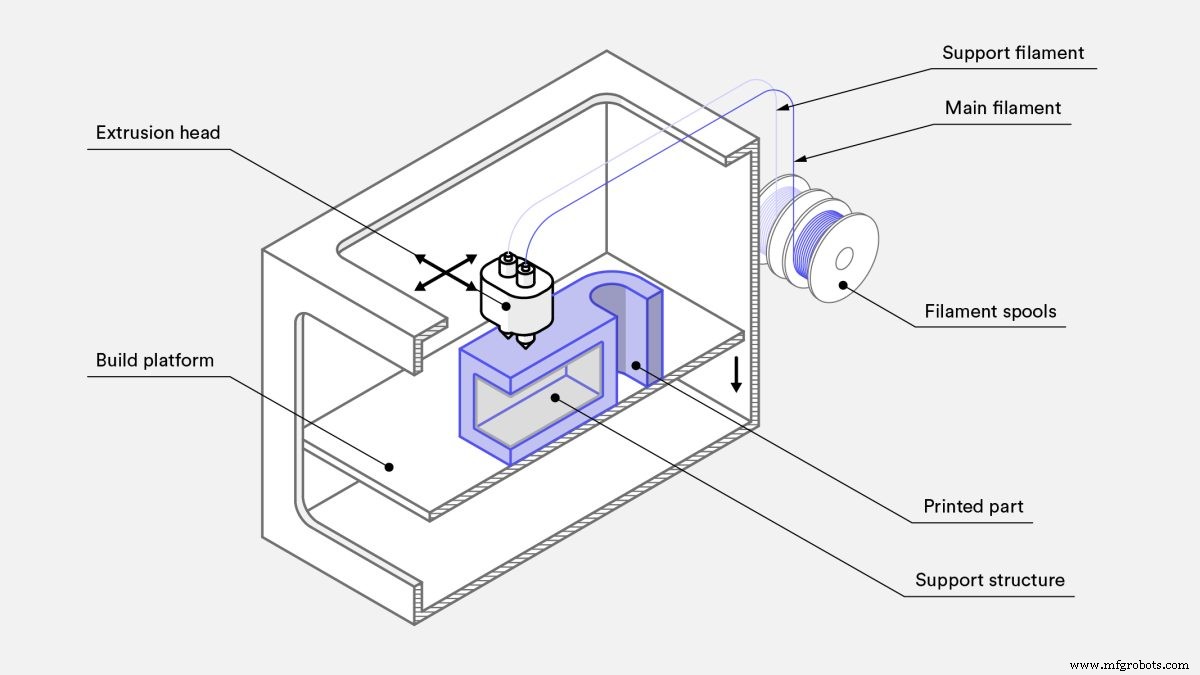
Qu'est-ce que l'extrusion de matériaux et comment ça marche ?
L'extrusion de matériaux produit des pièces en imprimant des couches de filaments thermoplastiques fondus les unes sur les autres. Une bobine de filament est alimentée à travers une buse d'extrusion chauffée et fondue. Les imprimantes déposent en continu le filament fondu à des endroits précis, où il refroidit et se solidifie. Imaginez un pistolet à colle chaude automatisé se déplaçant méthodiquement sur une surface de construction plane.
La buse de l'imprimante peut se déplacer le long de trois axes par rapport à la plate-forme de fabrication. Il trace la forme d'une seule coupe transversale de l'impression le long des axes x et y, superposant les coupes transversales les unes sur les autres le long de l'axe z pour créer l'impression complète.
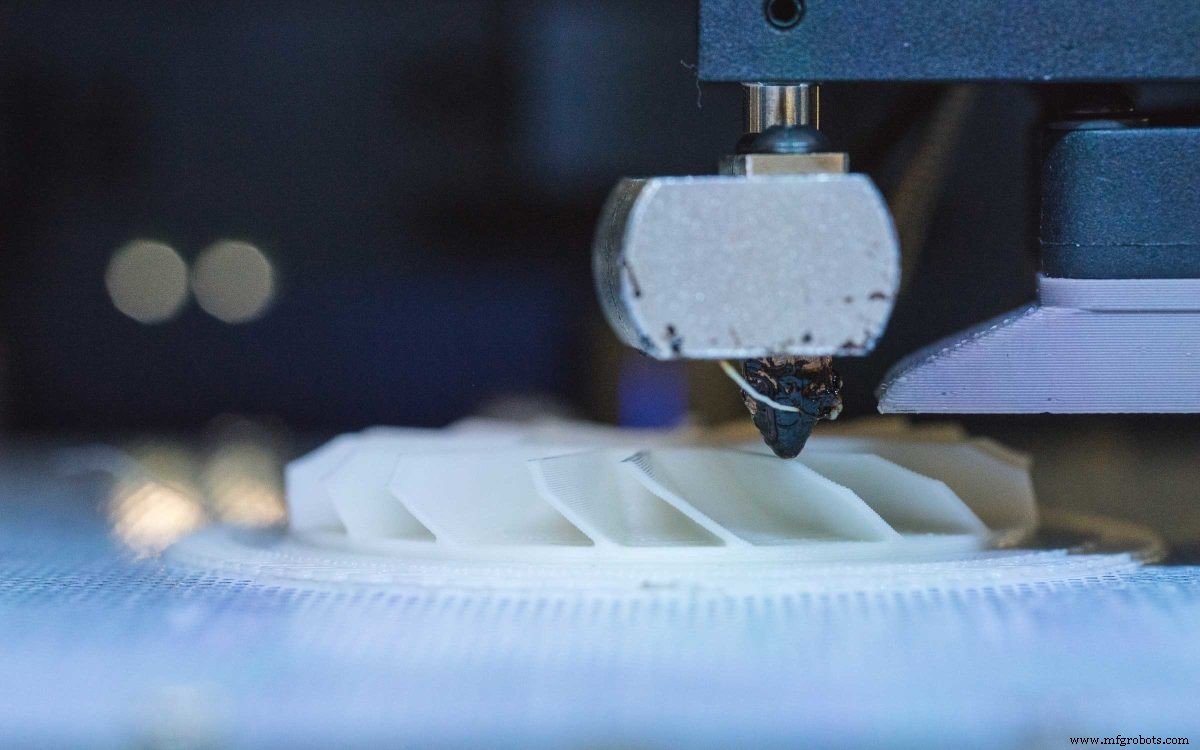
Types d'imprimantes d'extrusion de matériaux
La modélisation par dépôt de fil fondu (FDM) et la fabrication de filaments fondus (FFF) sont les deux types de technologies d'impression par extrusion de matériaux. L'extrusion de matériaux est relativement simple et est de loin la technologie d'impression la plus rentable et la plus largement disponible sur le marché.
Les deux principaux types de FDM sont le prototypage (desktop) et l'industriel. Nous différencions les deux parce que, bien que les technologies soient pratiquement identiques, l'échelle de chacune est très différente.
Matériaux pour l'extrusion de matériaux
Parce que le FDM est si largement utilisé, des milliers de filaments différents sont apparus sur le marché. Les plus courants sont de loin l'ABS et le PLA, mais le nylon, le PC, le PETG, le TPU et le PEI sont également disponibles. Certains filaments sont également renforcés de fibres, telles que le carbone, le Kevlar, la fibre de verre, le bois et le métal.
Pour plus d'informations sur la façon de choisir les bons filaments pour votre travail, nous avons rédigé un guide comparant différents matériaux d'impression 3D FDM .
Propriétés géométriques de l'extrusion de matériaux et FDM
Il existe des milliers de marques d'imprimantes FDM, de toutes formes et tailles. Les plus grands ont des plates-formes de construction d'environ 1 500 mm dans toutes les dimensions, tandis que les plus petits peuvent facilement tenir sur votre bureau à côté de votre machine à expresso.
Étant donné que les pièces sont construites de bas en haut, certaines caractéristiques, telles que les porte-à-faux, nécessitent des supports construits avec la pièce réelle. Cela étant, le FDM n'est pas toujours la solution idéale pour imprimer des composants plus complexes.
La résolution est fonction du filament, les filaments les plus fins ayant une épaisseur d'environ 0,15 mm. Le fait que le filament soit rond signifie que les angles vifs ne peuvent pas être imprimés et que les murs ne sont jamais plats à moins d'être traités après l'impression.
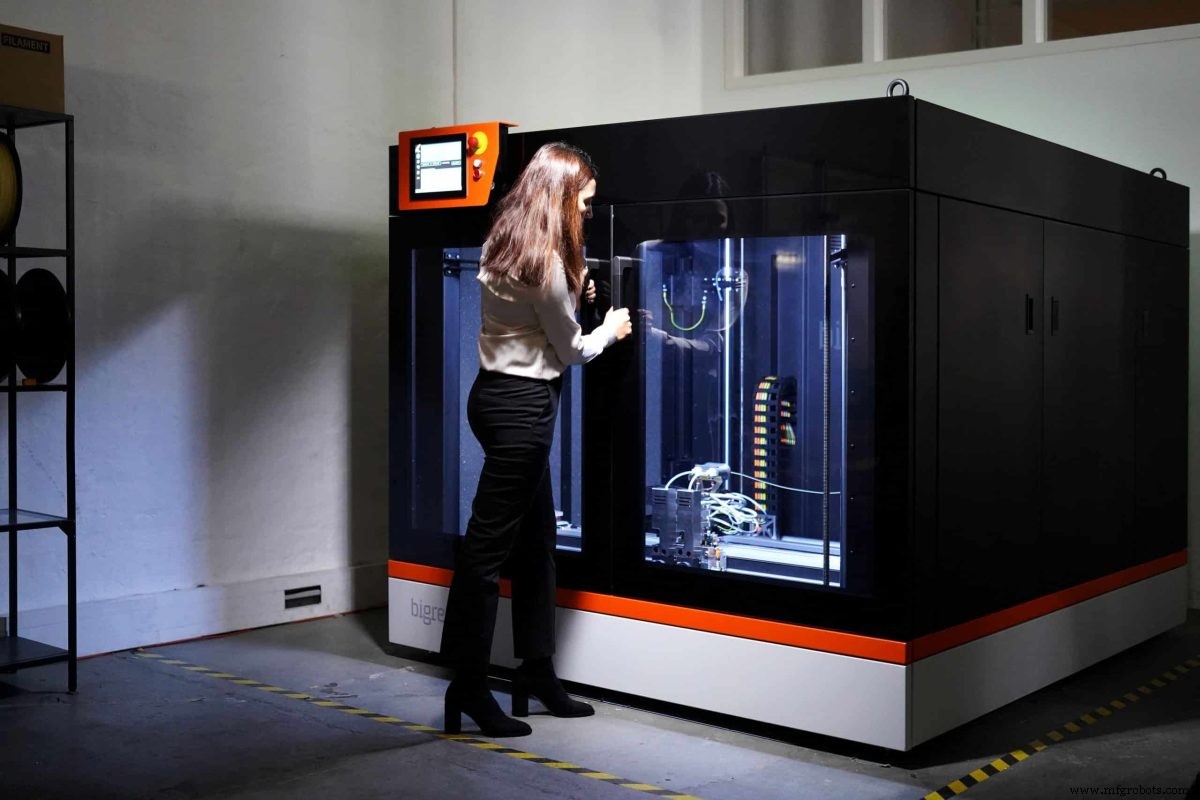
Propriétés mécaniques de l'extrusion de matériaux et FDM
Les imprimantes FDM ne sont généralement pas utilisées pour produire des pièces fonctionnelles d'utilisation finale, bien qu'il y ait quelques exceptions ici et là. Ce ne sont pas les imprimantes 3D les plus précises, car les pièces qu'elles impriment sont faibles le long de l'axe z. Les pièces ont également une superposition très claire sur chaque surface.
L'extrusion de matériaux et le FDM sont-ils rapides et rentables ?
Les imprimantes FDM sont connues pour être de niveau inférieur en termes de coût, les machines de bureau coûtant entre 500 et 5 000 dollars. Les machines de qualité industrielle, en revanche, peuvent être beaucoup plus chères et hors de portée financière, même pour les amateurs les plus dévoués.
Les machines d'extrusion de matériaux sont peu coûteuses à posséder et faciles à utiliser, mais l'externalisation est également populaire car l'omniprésence de la technologie rend les délais d'exécution d'un jour courants.
Les matériaux FDM standard sont largement disponibles et la concurrence maintient les prix bas. Les temps d'impression sont rapides pour les pièces unitaires mais, contrairement à la photopolymérisation en cuve ou PBF, il n'y a pas d'économies d'échelle. Cela rend le FDM relativement lent pour les gros volumes et n'offre probablement pas le meilleur rapport qualité-prix pour un grand nombre de pièces.
Applications commerciales :que pouvez-vous produire avec l'extrusion de matériaux et le FDM ?
Bien que les pièces FDM ne soient pas aussi durables ou esthétiques que les composants fabriqués à l'aide d'autres techniques, elles sont très rentables. Ces caractéristiques font de FDM la technologie la plus couramment utilisée pour de nombreuses étapes de prototypage, en particulier au début de la phase de preuve de concept.
La variété des matériaux disponibles, la rapidité et la rentabilité du FDM le rendent également très attractif pour certains types de pièces de production tant que la résolution et la finition de surface ne sont pas des facteurs critiques. Les imprimantes FDM industrielles peuvent facilement produire des prototypes fonctionnels et des pièces d'utilisation finale à partir de matériaux robustes, tels que des poignées, des gabarits et des fixations . Produire ces derniers composants avec des machines FDM est préférable à la fabrication traditionnelle en termes de prix.
Qu'est-ce que la fusion sur lit de poudre (PBF) et comment ça marche ?
Les imprimantes à fusion sur lit de poudre produisent des pièces en fondant ou en frittant sélectivement des particules de poudre pour former un objet entier. Le matériau en poudre est chauffé juste en dessous de son point de fusion et étalé sur la plate-forme de construction en une couche très fine. Un faisceau laser ou d'électrons est ensuite dirigé sur la surface de la poudre, fusionnant les particules pour former une seule coupe transversale de l'impression.
Après chaque couche, la plate-forme de construction est abaissée et le processus se répète. Chaque nouveau calque est fusionné au précédent jusqu'à ce que tous les calques aient été fusionnés en un seul objet.
Comme les couches sont construites les unes sur les autres, les particules non fusionnées agissent comme une structure de support pour l'impression, éliminant ainsi le besoin de la plupart des structures de support séparées. Une fois l'impression terminée, l'excédent de poudre de support est retiré et recyclé.
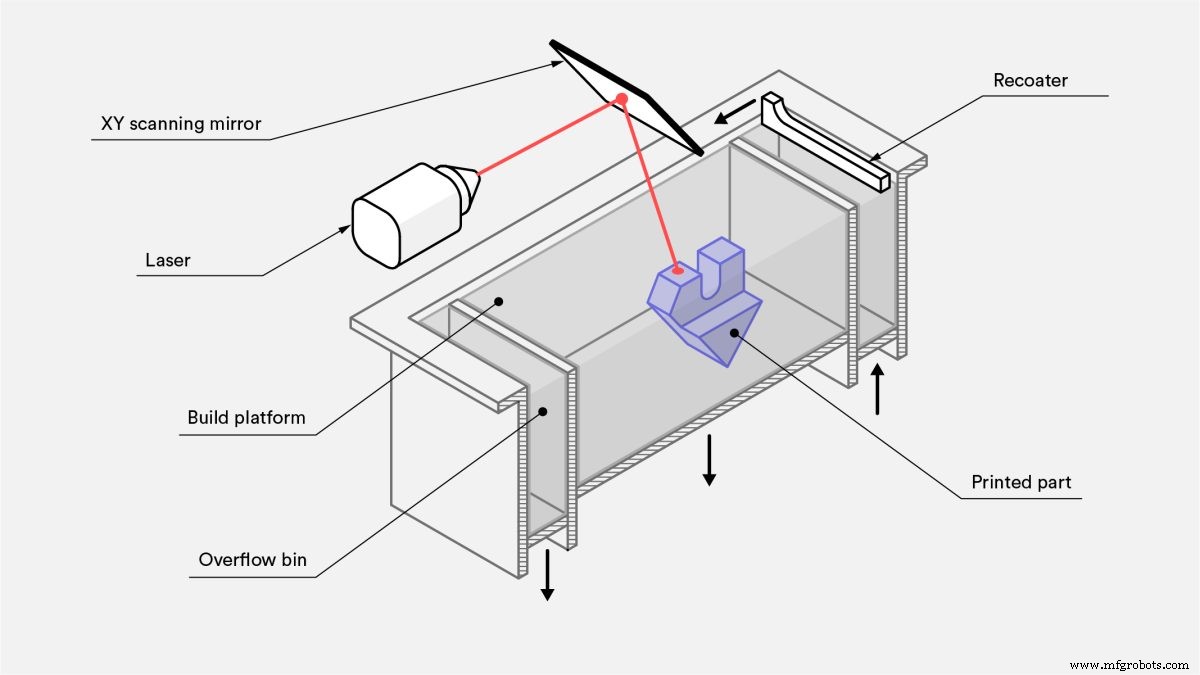
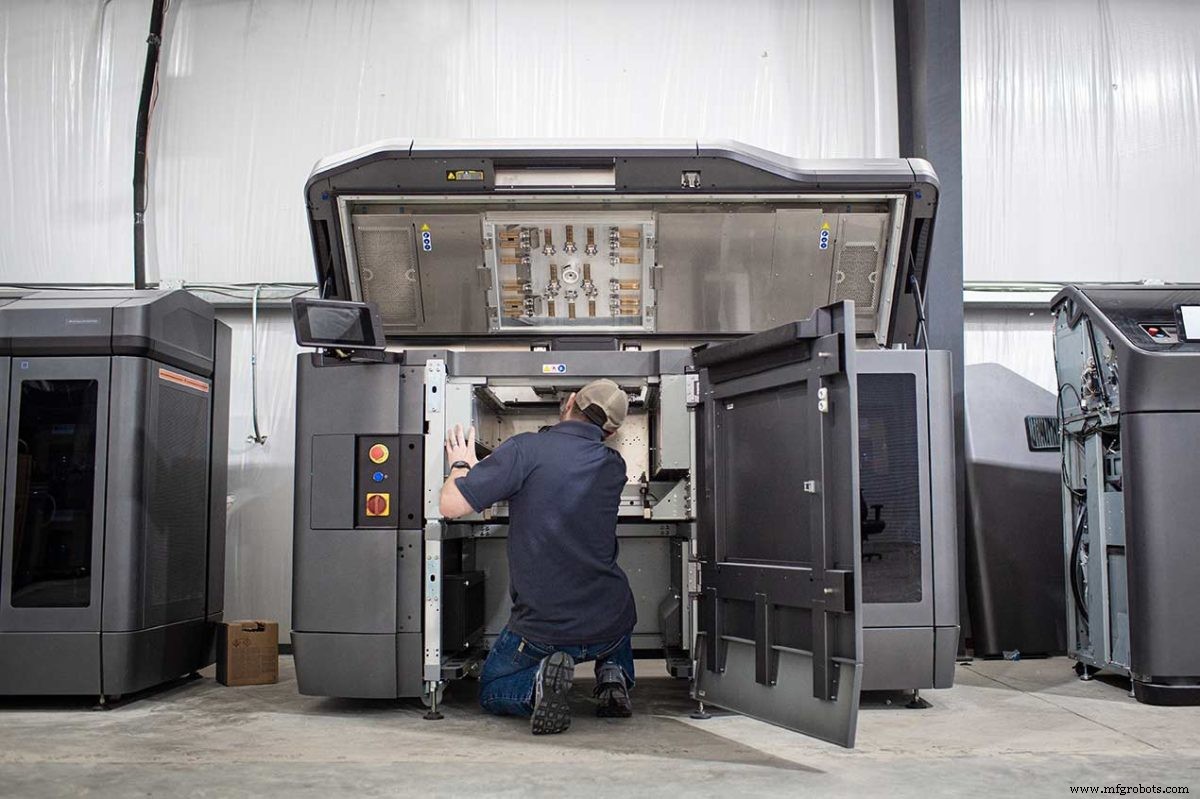
Types d'imprimantes PBF
De nombreuses imprimantes 3D utilisent la technologie PBF. Les types d'imprimantes les plus courants sont le frittage laser sélectif (SLS ), le frittage laser direct de métal (DMLS), la fusion laser sélective (SLM), la fusion multi-jets HP (MJF ), le frittage à grande vitesse (HSS) et la fusion par faisceau d'électrons (EBM). SLS est le plus courant pour les plastiques, et DMLS et SLM sont les plus courants pour les métaux.
-
Les imprimantes SLS produisent des pièces en plastique solides à l'aide d'un laser pour fritter les particules ensemble.
-
Les imprimantes DMLS créent des pièces métalliques poreuses à l'aide d'un laser pour fritter les particules ensemble.
-
Les imprimantes SLM produisent des pièces métalliques solides en utilisant un laser pour faire fondre les particules ensemble, pas seulement pour les fritter. Comme cela n'est possible que lorsque les particules ont le même point de fusion, SLM ne peut imprimer que dans des métaux simples, pas des alliages.
-
MJF produit des pièces en plastique solide grâce à une combinaison de technologies SLS et de projection de matériaux. Une fois qu'une couche de poudre plastique est étalée sur la plate-forme de fabrication, une tête d'impression avec des buses à jet d'encre dépose sélectivement des agents qui favorisent et inhibent la fusion. Un faisceau infrarouge haute puissance passe ensuite sur la couche, ne fusionnant que les zones où l'agent de fusion a été appliqué.
-
EBM produit des pièces métalliques solides en utilisant un faisceau d'électrons pour faire fondre les particules ensemble, mais les pièces doivent être produites sous vide. Le procédé ne peut être utilisé qu'avec des métaux conducteurs.
-
HSS utilise une tête d'impression à jet d'encre pour déposer un fluide absorbant les infrarouges directement sur une fine couche de granulés de plastique qui est étalée sur la surface chauffée d'une plate-forme de construction, délimitant la zone où le frittage est souhaité. La lumière infrarouge est ensuite utilisée pour fusionner la poudre sous le fluide en une couche.
-
MJF utilise une matrice à jet d'encre pour appliquer sélectivement des agents de fusion et de détail sur un lit de poudre de nylon, qui sont ensuite fusionnés par des éléments chauffants en une couche solide.
Matériaux pour la fusion sur lit de poudre
SLS prints in plastics and nylon composites, such as Alumide, which is a combination of nylon and aluminum. Polypropylene, a thermoplastic polymer, is also growing in popularity for both SLS and MJF.
While materials continue to evolve for SLS, nylon is still the most common material used. There are dozens of different nylons available, but PC, PEEK, PEKK, flexible TPUs, and composite and reinforced nylons are becoming common. Parts are usually printed in white and can be dyed any color.
MJF can only print in nylon. Because the fusing agent is black, in the past, parts could only be printed in gray. Today, however, HP Jet Fusion 500/300 series printers can print parts in full color and white. This series is being phased out but will still be supported for years.
Geometric properties of powder bed fusion
PBF printers can be manufactured larger than those for vat photopolymerization, but even large PBF printers rarely exceed 300 to 400 mm in any dimension. These printers can also print parts in high resolution. Because unused powder acts as a support material as print layers are built up, PBF can produce parts from very complex digital models.
Potential complexity is the same for MJF and SLS, with both achieving better results than SLA. However, SLA has even higher resolution (its layer height can go down to 25 microns whereas SLS always prints at 100 microns and MJF at 80 microns). MJF can produce slightly better resolutions than SLS, but SLS offers a broader range of materials
For metal, DMLS can print parts with some of the highest resolution available, followed by SLM and then EBM.
Mechanical properties of powder bed fusion
PBF can produce tolerances on par with vat photopolymerization, but PBF parts are much stronger. PBF can produce functional plastic parts with the best mechanical properties any 3D printing technology is capable of. MJF prints are slightly stronger than SLS and also have a smoother surface finish.
EBM systems produce less residual stresses than DMLS and SLM, resulting in less potential distortion. The metal parts made by DMLS are not as strong as those by SLM, since the powder particles are only sintered and parts remain slightly porous. However, SLM parts can have mechanical properties on par with traditional manufacturing technologies such as machining and forging.
All PBF prints have a slightly rough finish due to being made from powders, though can easily be polished smooth with simple post-processing.
Is powder bed fusion fast and cost-efficient?
PBF market competition continues to drive prices down, but it remains expensive. For metal 3D printing, it is still extremely expensive, usually costing more than CNC machining. For plastics, the cost is comparable to vat photopolymerization. MJF is usually around 10 percent cheaper than SLS.
When it comes to low volumes for plastics, SLS and MJF are slower than vat photopolymerization and FDM. However, they are the fastest for large batches because parts get printed directly on the build platform.
Commercial applications:what can you produce with powder bed fusion?
The ability to produce strong functional parts makes PBF the preferred technology for producing low volumes of functional plastic parts across all industries. Common applications include one-off industrial hardware such as machine parts, jigs, grips, and fixtures as well as low-volume production runs of customized plastic components.
Because of how expensive they are, DMLS, SLM, and EBM are only used when a part’s geometric complexity is too expensive to be machined or surpasses what machining can produce. Being able to produce very complex parts makes PBF the go-to technology for rapid prototyping.
What is material jetting and how does it work?
Material jetting produces parts by jetting tiny droplets of a liquid material onto the build platform and solidifying them with heat or light. Much like the standard 2D ink jetting process, a printhead with hundreds of material jets moves over a build platform, depositing material along an X-axis carrier, sweeping back and forth like the windshield wipers on a car and covering one whole layer in a single pass.
One printhead can carry jets for multiple materials, allowing for multi-material printing, full-color printing, and dispensing disposable support structures, such as wax.
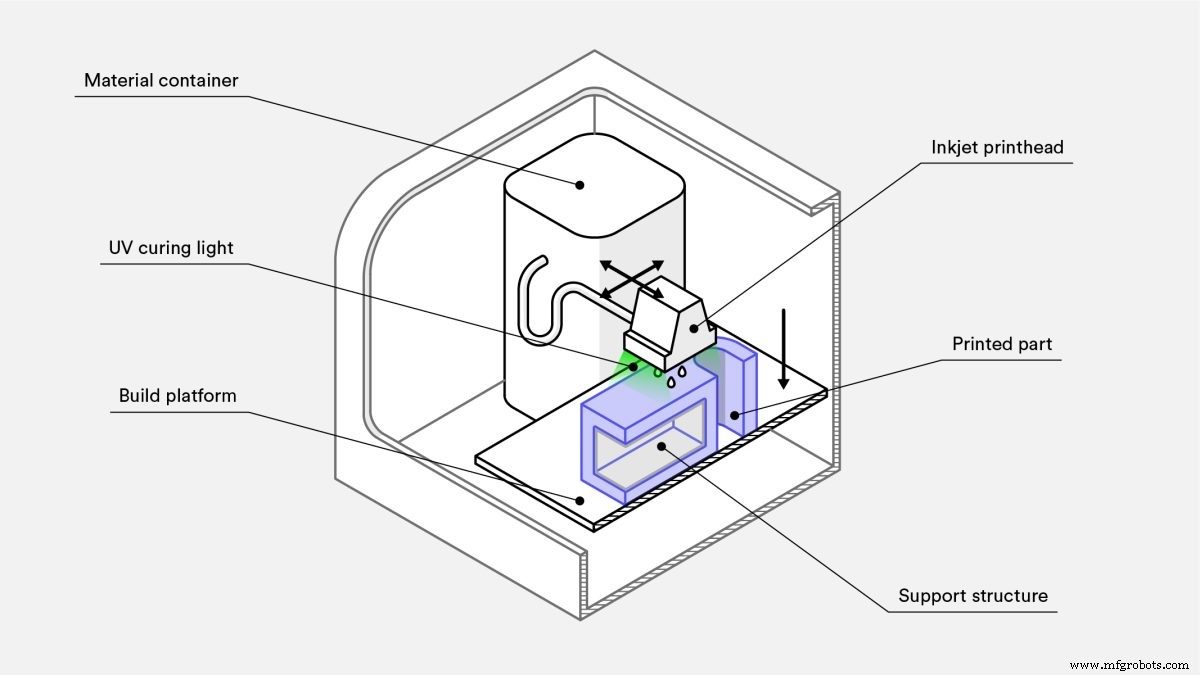
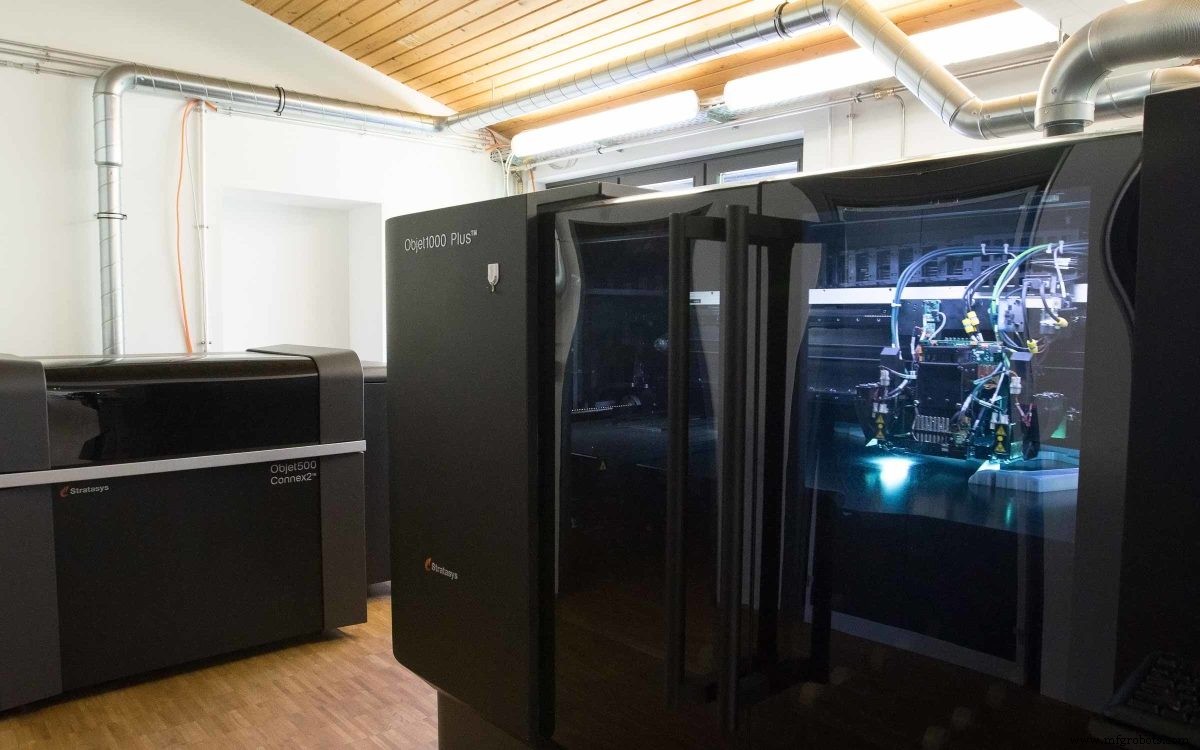
Types of material jetting 3D printers
Within the material jetting category there are a few distinct printing technologies. The three most common are PolyJet, NanoParticle Jetting (NPJ), and Drop-On Demand (DOD). As of mid-2022, PolyJet is by far the most popular.
-
PolyJet, the first material jetting technology, is patented and owned by Stratasys. This kind of printer dispenses liquid photopolymer resin and easy-to-remove support material from the printhead, which is then cured by a UV light.
-
NPJ is a technology patented by XJet. Unlike with PolyJet, where each layer is cured before the next is deposited, NPJ prints are cured once all the layers are deposited. Metal or ceramic nanoparticles are suspended in a liquid, which is deposited by the printhead along with support material. Heat in the printer causes the suspension liquid to evaporate as each layer is deposited, leaving only slightly bonded metal or ceramic and supports behind. Once the final layer is complete, the support material is removed and the whole part is sintered to bond all the nanoparticles.
-
DOD is a technology patented by SolidScape (acquired by Stratasys). It is very similar to PolyJet, but was developed specifically for high-precision printing in wax for investment casting and mold making, targeting the jewelry industry. Unlike in PolyJet, the printhead can print curves in high resolution by moving in both x- and y-axes. After the material droplets are deposited, each completed layer is skimmed with a fly cutter to ensure a perfectly flat surface. These factors improve the final dimensional accuracy.
Materials for material jetting
Due to the variety of technologies in the category, a wide range of materials is available for use with material jetting printers. The most common are photopolymers, flexible plastics, casting wax, metals, and ceramics.
PolyJet printers are known for being able to produce full-color, multi-material, multi-texture prints.
Geometric properties of material jetting
Build platforms can get quite large—measuring up to 1 square meter, they are almost as large as those used in FDM. Individual parts can also be very large, filling out the whole print bed. Given that fully solid supports are built up around the part, they can also produce very complex parts.
Mechanical properties of material jetting
Material jetting printers are highly precise and able to produce parts with very high tolerances, although the strength of parts is typically less than what FDM or PBF can achieve. The surface finishes are highly smooth, but there is also an option to print in a matte setting.
Is material jetting fast and cost-efficient?
No matter the printer type, material jetting is actually quite expensive. The materials are expensive and the support structures are printed solid, which means there is a lot of material wastage per part.
Production speeds are comparable with PBF printers.
Commercial applications:what can you produce with material jetting?
Material jetting is an expensive 3D printing technology, but its extremely high dimensional accuracy and smooth surface finishes make it the only viable solution when dimensional accuracy or impressive visuals are critical. This is often the case for highly realistic prototypes, anatomical models, complex and high-precision tooling, jewelry, medical devices, and surgical tools.
Multi-material printing is commonly used for haptic feedback prototypes, for example, a stiff case with flexible buttons.
What is binder jetting and how does it work?
Binder jetting produces parts by selectively depositing a binding agent over a powder bed. The build platform is first covered with a very thin layer of material powder. A printhead covered in inkjet nozzles then passes over, depositing a binding agent where the print is to be formed. Binder jetting printers can also print in color by depositing colored ink after the binding agent, before a new layer of powder covers the previous one.
Once the final layer is finished, the part is left to cure in the powder and let the binding agent gain strength. Once removed from the powder bin, some kinds of materials are ready. However, if parts are for functional use, most need to be infiltrated and sintered, causing them to shrink by up to 40 percent.
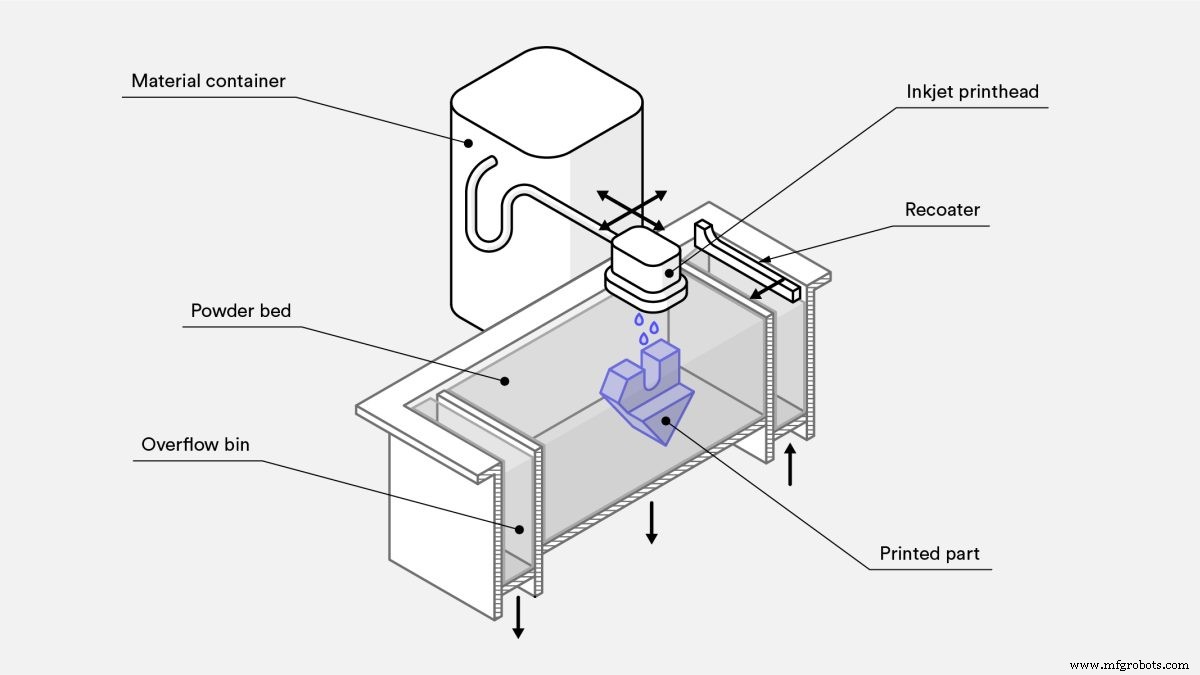
Types of binder jetting 3D printers
Binder jetting is achieved by only one technology, though there are many different kinds of printers, differentiated by the kinds of materials and binders they can use.
To learn more, read our introductory article on binder jet 3D printing.
Materials for binder jetting
Because prints are held together by a binding agent—as opposed to bonds between particles of the build material—many materials that can be powdered can be printed with binder jetting. The most common materials are sands, ceramics, and metals, though plastics can also be used.
Geometric properties of binder jetting
Binder jetting is best suited for parts smaller than the size of a fist.
Because the unused powder serves as a natural support structure, complex parts are also possible. However, one constraint is that thickness should never exceed 10mm because the filtration may be inconsistent. As well, the resolutions are high, on par with PBF.
Mechanical properties of binder jetting
Binder jetting can produce parts with good tolerances, but the final tolerance can be hard to predict since shrinkage occurs with post-processing.
Before infiltration, metal parts are extremely weak and can crumble if not handled carefully. After infiltration, they are close to fully dense, but their mechanical properties do not meet the higher quality of traditionally manufactured parts.
The surface roughness of these metal parts is better than that achieved with DMLS and SLM.
Is binder jetting 3D printing fast and cost-efficient?
Binder jetting is an affordable technology, costing even less than vat photopolymerization and PBF. Print speeds are comparable to PBF and in line with other technologies for low-volume runs, but fast for higher volumes.
Commercial applications:what can you produce with binder jetting?
Because it can produce complex parts quite quickly and cost-effectively in a variety of colors, binder jetting is ideal for full-color prototyping. The price point of binder jetting is lower than material jetting and, despite its mechanical-property limitations, it can still achieve resolutions suitable for most prototypes.
This is also one of the fastest and most affordable techniques for producing complex, high-precision metal and ceramic parts. Many different powders are available, and print beds can be relatively large. The mechanical properties of binder-jetted metal parts do not match the strength or tolerances of PBF prints but they can still be functional if infiltrated and sintered.
Binder jetting is especially attractive for producing complex casts from sand because it can print large, complex geometries at a relatively low cost. And the process is simple enough to be integrated with most traditional foundry processes.
What is direct energy deposition and how does it work?
Direct energy deposition (DED) produces parts by layering beads of molten material, which is usually metal. The technology is very similar to that used in plastic material extrusion printers, but for metal. The feedstock material, which is either powder or wire, is continuously pushed through a nozzle and melted by a laser or an electron beam or arc at the point of deposition, where it cools and solidifies.
The nozzle can move along multiple axes in relation to the build platform. Three-axis machines trace the shape of a single cross-section of a print along the x-axis and y-axis, layering cross-sections on one another along the z-axis to build up the full part. Five-axis DED printers are not limited to building up parts layer by layer because they can deposit material from any angle. This means they can do more than simply build up parts from scratch and are often used to deposit material on multiple sides of existing objects.
DED is often referred to as direct metal deposition (DMD). There are also many different proprietary technologies whose names are often used interchangeably, even though they differ in their materials and energy sources.
All work according to very similar principles. Laser Engineered Net Shaping (LENS) by Optomec is the best-known example of a technology that fuses powders with a high-powered laser. Electron Beam Additive Manufacturing (EBAM) by Sciaky is the best-known example of a technology that fuses extruded wire with an electron beam.
Types of DED printers
LENS utilizes a deposition head consisting of a laser head, powder dispensing nozzles, and inert gas tubing. The laser travels through the center of the head to create a melt pool on the build area and powder is sprayed from the sides where it is melted and then solidified. The inert gas forms an oxygen- and moisture-free shroud, which prevents surface oxidation and promotes better layer adhesion.
EBAM feeds metal welding wire through the nozzle and melts it with an electron beam at the contact point with the build area. Essentially, EBAM is FDM for metals.
Materials for DED
LENS technology can print in both metals and ceramics, although ceramics are by far the more common material in use. Available materials for both LENS and EBAM include almost any weldable metal, such as aluminum, steel, titanium, Inconel, tantalum, tungsten, nickel, and niobium.
Geometric properties of DED
Another key advantage of DED is the very large print bed sizes. It is not uncommon for large manufacturers to build customer DED printers with build envelopes that are multiple meters long along any dimension.
Support structures are possible but difficult because the large liquid melt pool at the deposition point does not allow for overhangs. The same attribute means complex geometries are also not possible. Resolution is very poor compared with other metal 3D printers. Powder particle sizes are between 50 and 150 microns and welding wire ranges from 1 to 3mm in diameter.
Sharp corners, for example, can only be achieved in post-processing, typically by a CNC mill. This is very common practice, as new material is built up with DED and then machined to the desired precision.
Mechanical properties of DED
The high amount of energy required to maintain a melting point at the point of deposition creates large thermal gradients that can cause a lot of residual stress, but DED produces fully dense parts with mechanical properties that are as good as forged metal parts.
The low resolution, however, means parts tend to have a poor surface finish, requiring secondary machining to achieve the most desired results.
Is DED fast and cost-efficient?
The two most important advantages of DED are print speed and material cost. All DED technologies are relatively fast at laying down material, with the fastest machines printing 11kg per hour, (albeit with very low resolution). The metal feedstock used is also cheaper than that used by other metal 3D printers.
The powders are usually commercial off-the-shelf (COTS) materials designed for welding and are widely available. But for simple parts, traditional manufacturing is still almost always cheaper.
Commercial applications:what can you produce with DED?
The three main applications of DED are part repairs, feature additions, and near-net-shape part production. Essentially a form of welding, DED can print onto existing parts. This makes it ideal for repairing broken parts and adding features that cannot be added via other processes. Tool repair is by far the most common use, and companies turn to DED when expensive machinery proves more cost-effective to fix than reorder, such as in heavy industry.
Because the resolutions are so low, most parts also require post-processing with a CNC mill. Parts are therefore printed near to net shape, with the expectation that they will be machined to proper tolerances. Because conventional manufacturing is almost always cheaper, near-net-shape DED printing is only used when traditional manufacturing is very slow, expensive, or simply not possible.
What is sheet lamination and how does it work?
Sheet lamination produces parts by stacking and laminating sheets of material cut to match a part’s single-horizontal cross-sections. In some printers, the sheets are first cut and then laminated. In most, the sheets are first laid and laminated and then cut to size.
Types of sheet lamination printers
This is one of the simplest methods of building up 3D models. Despite its simplicity, there are many different proprietary technologies based on material, lamination method, and cutting method. In most cases, the process is a simple variation of paper laminated object manufacturing (LOM). Ultrasonic consolidation (UC) is the only radically different technology, as it uses ultrasonic welding rather than a separate bonding agent.
-
LOM laminates sheets together with a bonding adhesive and then subtracts features layer by layer, using CNC milling, laser cutting, or water-jet cutting.
-
UC follows the same process at LOM, except the lamination is achieved through ultrasonic vibrations as a form of friction welding.
Materials for sheet lamination
Across all the different types of printers, there are many available materials:papers, most polymers, fiber-reinforced polymers, ceramics, and just about any metal. Multi-material layers can also be achieved with all these materials, provided each layer can be laminated and shaped with the same methods.
Using colored sheets enables full-color prints across the color spectrum.
Geometrics properties of sheet lamination
Sheet lamination print beds vary quite a lot in size, but are comparable to SLA and SLS printers. Large-format printers are not common.
Because the sheet cutting methods are relatively simple, highly complex shapes are not possible. However, because support structures are not necessary, internal structures are possible.
One additional design option is to lay embedded wiring between sheets. Most processes do not require heat, so expect a reduced risk of high temperatures destroying them. Typical layer resolution depends entirely on the material feedstock
Mechanical properties of sheet lamination
Dimensional accuracy and surface finishes are on par with what can be achieved with a simple CNC milling machine, laser cutter, or water-jet cutter. The weakness of the bond between sheets, however, means that these parts are unsuitable for structural or functional purposes.
Is sheet lamination fast and cost-efficient?
LOM is very cost-effective thanks to the ready availability of all the raw materials. The lack of pre-production preparation means the printers are also very fast.
What are the commercial applications of sheet lamination?
LOM was originally used in architecture for building models. Today its most common use is for highly detailed, colored objects, typically for proof-of-concept and look-and-feel prototyping.
Frequently asked questions
How do I decide the right 3D printing technology for my custom parts?
There are many factors to think about before settling on the right 3D printer for your manufacturing needs. These include functionality, materials required, cosmetic characteristics, process capabilities and many more. To start, check out this comprehensive resource on selecting the right 3D printing process, and remember that you can (and should) always test different printing methods before finding the one that's perfect for your custom parts.
What 3D printer is optimal for complex geometries.
For designs with complex geometries, we highly recommend opting for a powder bed fusion technology like SLS or MJF.
What do I do if several 3D printers work for my custom parts?
Oftentimes, several different 3D printing processes can produce your parts. If this ends up being the case, compare the cost and properties of each to determine the right manufacturing fit.
What 3D printing technology is right for functional polymer parts?
For functional polymer parts, go for thermoplastics ( SLS or FDM) over thermosets (SLA/DLP or material jetting).
What 3D printing technologies will give me the best cosmetic appearance?
For the best cosmetic quality, thermosets (SLA/DLP or material jetting) will give you the most impressive aesthetics.
What's the best 3D printing technology for metal parts?
Honestly, we recommend pivoting to CNC machining for producing metal parts, though if your designs are very complex, DMLS and SLM are viable alternatives to milling and turning.
impression en 3D
- Que sont les technopolymères et à quoi servent-ils ?
- Quels sont les différents types d'ongles ?
- Les nombreux types de polyuréthane et leur utilisation
- Quels sont les types d'actionneurs
- Quels sont les types de sécheurs à air comprimé ?
- Quels sont les types courants d'échelles de maintenance ?
- Quels sont les différents types de cintrage de tubes ?
- Quels sont les différents types d'agents d'entretien ?
- Excavatrices :description et types pouvant vous aider dans votre projet.